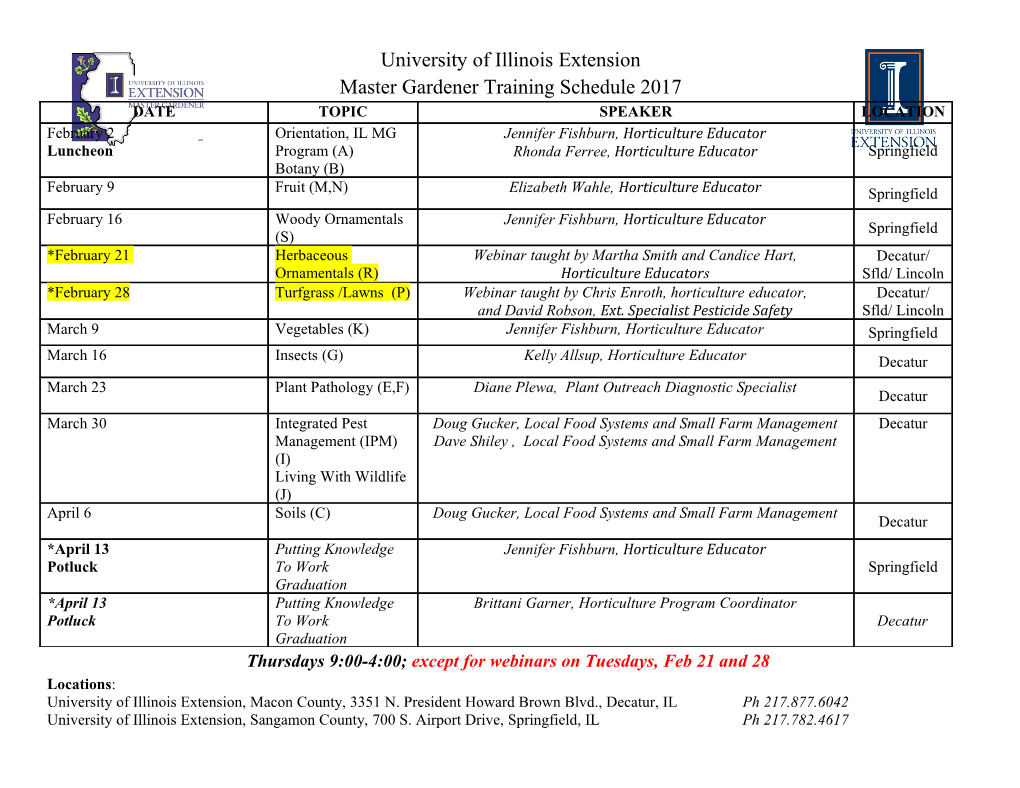
Bringing Optical Metamaterials to Reality By Jason Gage Valentine A dissertation in partial satisfaction of the requirements for the degree of Doctor of Philosophy in Engineering – Mechanical Engineering in the Graduate Division of the University of California, Berkeley Committee in charge: Professor Xiang Zhang, Chair Professor Costas Grigoropoulos Professor Liwei Lin Professor Ming Wu Fall 2010 Bringing Optical Metamaterials to Reality © 2010 By Jason Gage Valentine Abstract Bringing Optical Metamaterials to Reality by Jason Gage Valentine Doctor of Philosophy in Mechanical Engineering University of California, Berkeley Professor Xiang Zhang, Chair Metamaterials, which are artificially engineered composites, have been shown to exhibit electromagnetic properties not attainable with naturally occurring materials. The use of such materials has been proposed for numerous applications including sub-diffraction limit imaging and electromagnetic cloaking. While these materials were first developed to work at microwave frequencies, scaling them to optical wavelengths has involved both fundamental and engineering challenges. Among these challenges, optical metamaterials tend to absorb a large amount of the incident light and furthermore, achieving devices with such materials has been difficult due to fabrication constraints associated with their nanoscale architectures. The objective of this dissertation is to describe the progress that I have made in overcoming these challenges in achieving low loss optical metamaterials and associated devices. The first part of the dissertation details the development of the first bulk optical metamaterial with a negative index of refraction. This metamaterial is shown to overcome the problems of previous metamaterials by reducing the amount of light absorbed in the material. The increased thickness of the bulk metamaterial also allows the first direct experimental observation of negative refraction at optical frequencies. Next, I will describe the design and experimental realization of the first electromagnetic cloak operating at optical frequencies. The cloaking device is designed using quasi-conformal mapping which enables the use of an all dielectric, isotropic metamaterial, allowing the cloak to operate over large bandwidth with low absorption losses, overcoming the problems with previous cloaking proposals. This design methodology and metamaterial system is then extended to realize an optical ‘Janus’ device. The device is designed and experimentally proven to have two different and independent optical functionalities in two separate spatial directions for use in integrated photonics architectures. The last section of the thesis describes several new directions I am pursuing in the field of metamaterials. The first is the experimental realization a photonic black hole. This device functions similar to a gravitational black hole in that it concentrates light into a small spatial area but is realized through a spatial varying index profile. The extension of transformation optics into plasmonic systems is then presented. Experimental work aimed 1 at realizing a gradient index Luneburg lens is presented utilizing modulations of the surface plasmon mode index though height variations in a dielectric. Finally, a new method for achieving large scale, three dimensional, gradient index metamaterials is presented. Such a method could be employed for achieving large scale cloaks or gradient index lenses for use in photovoltaics. The findings presented here represent some of the first metamaterial inspired devices at optical wavelengths. It is my hope that this work will help to inspire the next generation of metamaterials and devices with ever increasing functionality. 2 To my loving parents, without your support none of this would have been possible. i Table of Contents Table of Contents ii List of Figures iv Acknowledgements xiii Chapter 1 Metamaterials 1 1.1 Metamaterials: What is all the fuss about? 1 1.2 Engineering Material Properties 3 1.2.1 Effective Media 5 1.2.2 Negative Refractive Index 7 1.3 Optical Metamaterials 11 1.3.1 Cut-Wire 12 1.3.2 Fishnet 13 1.4 Organization of the Dissertation 15 References 16 Chapter 2 Bulk Optical Negative Index Metamaterial 20 2.1 Introduction 20 2.2 Bulk Fishnet Design 21 2.3 Fishnet Fabrication 25 2.3.1 Focused Ion Beam Milling 26 2.4 Experimental Results and Discussion 29 2.5 Conclusions and Outlook 34 References 34 Chapter 3 Broadband Optical Cloaking 37 3.1 Transformation Optics 37 3.1.1 Electromagnetic Cloaking 38 3.2 Carpet Cloak Design 40 3.3 Carpet Cloak Fabrication 44 3.4 Experimental Results and Discussion 47 3.5 Conclusions and Outlook 52 References 53 Chapter 4 Janus Device 56 4.1 Introduction 56 4.2 Janus Device Design 57 4.3 Janus Device Fabrication 59 4.3.1 Reactive Ion Etching 59 4.4 Experimental Results and Discussion 62 4.5 Conclusions and Outlook 67 References 67 ii Chapter 5 New Directions in Metamaterials 70 5.1 Photonic Black Holes 70 5.2 Plasmonic Transformations 75 5.3 Scalable 3D Manufacturing 82 5.4 Conclusions and Outlook 86 References 86 Chapter 6 Conclusion and Outlook 89 6.1 Conclusions 89 6.2 The Future of Metamaterials 90 iii List of Figures Figure 1.1. Material parameter space. Optical materials are characterized by the electric permettivity (ε ) and the magnetic permeability ( μ ). 4 Figure 1.2. Field ratios for effective medium of different shaped constituents. (a) spherical and (b) cylindrical inclusions. These ratios are used in equation 1.5 to compute the effective material properties for both types of constituents where ε e and εi are the matrix and inclusion permittivity, respectively. 6 Figure 1.3. Left and Right handed triads. (a) While positive index materials are characterized by energy (S) and phase (k) moving in the same direction, these vectors move in opposite directions (b) in negative refractive index materials. 7 Figure 1.4. Effective permittivity of a Silver wire composite medium for various filling fractions. The wave is polarized along the wire axis leading to a non- resonant diluted metal. Tuning the filling fraction allows engineering of the effective permittivity. 8 Figure 1.5. Schematic of the split-ring resonator. (a) Depicts an array of the resonators and the proper polarization of light needed to create an effective magnetic response (b) Unit cell of the metamaterial with annotated dimensions where P is the periodicity of the array. (c) Equivalent circuit of the split-ring where at resonance the internal current (j) can induce a magnetic field that is out of phase with the incident light. 9 Figure 1.6. Permeability of the split-ring metamaterial. The resonator passes through resonance at ~1Ghz wherein a negative effective permeability, Re(µ) can be realized. This resonance is accompanied by a loss, Im(µ), due to the finite conductivity of the metal. 10 Figure 1.7. Evolution of the split-ring unit cell as frequencies scale to the optical regime. 12 Figure 1.8. Cut-wire schematic. (a) Depicts an array of the cut-wires which can lead to a negative index of refraction under the proper polarization. (b) Unit cell of the cut-wire metamaterial where the grey and green wires are made of metal and dielectric, respectively. (c) Circuit model of the cut-wire unit cell depicting the internal LC circuit and current loop. 13 Figure 1.9. Fishnet metamaterial schematic (a-c) Depict the separate components of the metamaterial which provide both a magnetic (a) and electric (b) response. The silver layers are made of metal and the green layer a dielectric. Combined (c), these constituents form the complete fishnet structure. (d) Unit cell geometry of the metamaterial. (e) Depicts the LC circuit and current loop that form the iv magnetic response in the fishnet metamaterial. 14 Figure 2.1. Schematic and electromagnetic response of a single layer fishnet. (a) Schematic of the fishnet design and incident polarization where the dotted line indicates the cross-section of the material analyzed in (b,c). (b) Equivalent circuit of the NIM showing the induced current loop in the material. (c) Finite difference time domain simulation of the electric field within the magnetically resonant portion of the fishnet. The high electric field inside the metal layers is indicated by the strong red / blue color in the electric field plot. The high field, and resulting current, results in absorption of light due to the losses in the metal. 21 Figure 2.2 Schematic of the bulk fishnet metamaterial. (a) Schematic of 3 functional layers of a bulk fishnet along the direction of illumination. (b) Unit cell of the fishnet with annotated dimensions. The grey color corresponds to Silver while the green corresponds to Magnesium Fluoride. 22 Figure 2.3 Dispersion relations and field plots bulk fishnet structure. In all plots the grey area corresponds to the negative material property regions as determined by simulations. (a) Dispersion relation of the array of metal strips along the magnetic field. The grey area indicates the bandgap where µ < 0. (b) Dispersion relation for the array of metal wires aligned along the electric field. The grey area indicates the region where ε < 0. (c) The dispersion for the 3D fishnet structure. A dispersion curve with negative slope appears (grey region) within the overlapped region of the electric band gap and magnetic band gap if both structures are combined. (d) Magnetic field plot below the band gap. (e) Magnetic field plot above the band gap. The dotted lines in the schematic mark the unit cell size. 23 Figure 2.4 Consequences of coupled layers in the bulk fishnet metamaterial. (a) Schematic of a 3 functional layer fishnet showing the cross-section taken in (b,c). (b) Depiction of the cascaded circuit which leads to the magnetic response of the structure. Notice that the current flow on the top and bottom surface of the metal is out phase. (c) Electric field plot of the fishnet generated with FDTD. It can be observed that indeed, the electric field, on the top and bottom of the metal surfaces is out of phase.
Details
-
File Typepdf
-
Upload Time-
-
Content LanguagesEnglish
-
Upload UserAnonymous/Not logged-in
-
File Pages108 Page
-
File Size-