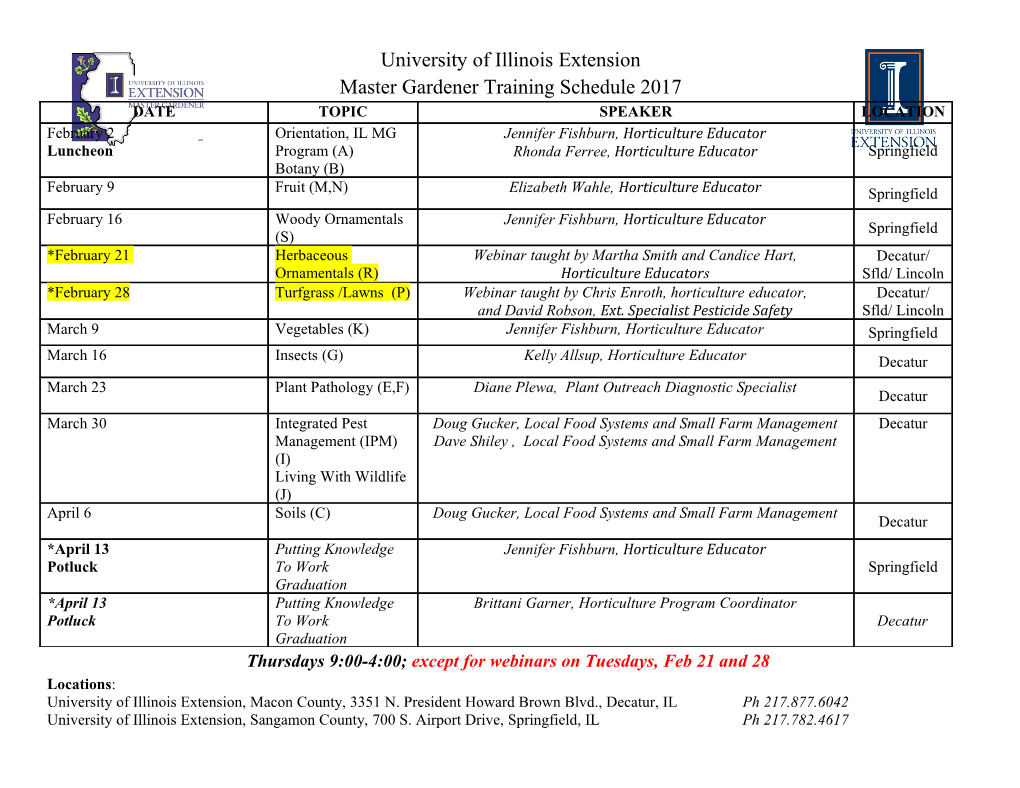
Vasily D. Sumanov1, Dmitry А. Aksyonov2, Oleg A. Drozhzhin1,2, Igor A. Presniakov1, Alexey V. Sobolev1, Iana S. Glazkova1, Alexander A. Tsirlin3, Dmitry Rupasov2, Anatoliy Senyshyn4, Irina V. Kolesnik5,6,1, Keith J. Stevenson2, Evgeny V. Antipov1,2, Artem M. Abakumov2* 1 Department of Chemistry, Lomonosov Moscow State University, 119991 Moscow, Russian Federation 2 Skoltech Center for Electrochemical Energy Storage, Skolkovo Institute of Science and Technology, Nobel str. 3, 143026 Moscow, Russian Federation 3 Experimental Physics VI, Center for Electronic Correlations and Magnetism, Augsburg University, 86159 Augsburg, Germany 4 Forschungsneutronenquelle Heinz Maier-Leibnitz (FRM II), Technische Universität München, Lichtenbergstrasse 1, 85747 Garching, Germany 5 Department of Materials Science, Lomonosov Moscow State University, 119991 Moscow, Russian Federation 6 Kurnakov Institute of General and Inorganic Chemistry RAS, 119991 Moscow, Russian Federation ABSTRACT: Lithium iron phosphate LiFePO4 triphylite is now one of the core positive electrode (cathode) materials ena- bling the Li-ion battery technology for stationary energy storage applications, which are important for broad implementa- tion of the renewable energy sources. Despite the apparent simplicity of its crystal structure and chemical composition, LiFePO4 is prone to off-stoichiometry and demonstrates rich defect chemistry owing to variations in the cation content and iron oxidation state, and to the redistribution of the cations and vacancies over two crystallographically distinct octa- hedral sites. The importance of the defects stems from their impact on the electrochemical performance, particularly on limiting the capacity and rate capability through blocking the Li ion diffusion along the channels of the olivine-type LiFePO4 structure. Up to now the polyanionic (i.e. phosphate) sublattice has been considered idle on this playground. Here, we demonstrate that under hydrothermal conditions up to 16% of the phosphate groups can be replaced with hy- droxyl groups yielding the Li1-xFe1+x(PO4)1-y(OH)4y solid solutions, which we term “hydrotriphylites”. This substitution has tremendous effect on the chemical composition and crystal structure of the lithium iron phosphate causing abundant population of the Li-ion diffusion channels with the iron cations and off-center Li displacements due to their tighter bonding to oxygens. These perturbations trigger the formation of an acentric structure and increase the activation barri- ers for the Li-ion diffusion. The “hydrotriphylite”-type substitution also affects the magnetic properties by progressively lowering the Néel temperature. The off-stoichiometry caused by this substitution critically depends on the overall con- centration of the precursors and reducing agent in the hydrothermal solutions, placing it among the most important pa- rameters to control the chemical composition and defect concentration of the LiFePO4-based cathodes. with dedicated morphology, it delivers outstanding rate capability maintaining up to 90% of the discharge capaci- Starting from the first report in 1997 by Padhi et al. on ty at 10C rate.3-8 Because of its great technological im- electrochemical activity of the LiMPO4 (M – 3d transition portance, crystallography and defect chemistry of metal) phospho-olivines as positive electrode (cathode) LiFePO4 has been carefully scrutinized by most of the materials for Li-ion batteries,1 lithium iron phosphate available experimental and computational techniques, LiFePO4 (also known as the mineral triphylite) has be- also in relation to the synthesis conditions and post- come a commercially sold material with the annual pro- synthesis treatments. duction volume of thousands metric tons.2 This material The crystal structure of LiFePO4 is based on a distorted is environmentally benign and economically more viable hexagonal close packing of the oxygen atoms, where the than layered oxide cathodes as it does not contain toxic Li+ and Fe2+ cations reside in octahedral interstices (de- and expensive cobalt, being also safer due to the tight noted as the M1 and M2 positions, respectively), whereas binding of oxygen into the phosphate groups. Moreover, the P5+ cations occupy 1/8 of the tetrahedral voids. The it offers high practical capacity of ~160 mAh/g and the + FeO6 octahedra and PO4 tetrahedra form a framework reasonably high operation voltage of 3.4V vs. Li/Li . When with the channels running along the [010] direction of the prepared in the form of nanosized carbon-coated particles Pnma LiFePO4 structure, serving as one-dimensional (1D) LiFePO4, and phosphorous deficiency up to 16% can be diffusion pathways for the Li+ cations. Despite its appar- achieved. This replacement appears as an important fac- ent simplicity, LiFePO4 demonstrates very rich defect tor, tightening Li binding to the oxygen atoms and in- chemistry that still remains largely controversial. The M1 creasing the diffusion barriers, introducing more Fe into and M2 sites adopt a distorted octahedral oxygen coordi- the M1 position, causing Li deficiency and, eventually, nation with very similar average metal-oxygen distances altering crystal structure, electrochemical and magnetic of 2.150 and 2.156Å, respectively,9,10 that together with the properties. closeness of the Li+ (r = 0.76Å) and Fe2+ (r = 0.78Å)11 ionic radii promotes antisite disorder between the M1 and M2 ' sites leading to the FeLi Li Fe defect pairs (denoted with the Kröger-Vink notations). The formation of these antis- H3PO4, LiOHH2O, FeSO47H2O and ascorbic acid taken ite defects has been confirmed by numerous diffraction in the 1:3:1:1 molar ratio were used as initial reagents. All 12-16 studies, and directly observed by transmission electron reagents were purchased from Sigma Aldrich (puri- 17 microscopy. Computational methods identify them as ty>99%) and were used without any preliminary treat- the lowest-energy and, therefore, most abundant defects ment. Water content in FeSO 7H O was checked via the 18 4 2 in LiFePO4. These defects are deemed the major obstacle + thermogravimetric method. First, FeSO4 7H2O, H3PO4 for the reversible Li (de)intercalation from LiFePO4, be- 2+ and ascorbic acid were dissolved in distilled water. After- cause even a relatively small fraction of the Fe cations wards, LiOH aqueous solution was added dropwise to the residing in the [010] channels effectively blocks the diffu- reaction mixture under continuous stirring. Then the 50 sion pathways rendering part of the lithium cations elec- ml (except for the samples #1 and #2) hydrothermal trochemically inactive and thus reducing the capacity,19 PTFE-lined reactor was purged with N2 gas, sealed and although alternative diffusion paths with relatively low thermally treated according to the protocol listed in Table activation barriers around the antisite defects may be 1. possible.20 The antisite disorder is not the only source of The #1 and #2 samples were synthesized by the original Fe in the M1 position. For example, the material can be hydrothermal technique developed to produce larger doped with iron at the cost of Li vacancies in order to batches (2 and 3.5 g, respectively) of single-phase triphy- maintain the charge balance, and the Fe' V defect Li Li lite material at very low solution concentrations. The pair forms.21,22 Another degree of freedom is due to a small 3+ 21 main differences from the majority of procedures report- fraction of Fe introduced during the synthesis. By com- ed in the literature were zero time of the hydrothermal bining all these possibilities, rather complex off- soaking period at the target temperature point, as well as stoichiometric compositions with various fractions of Li, the fast heating (~4 oC/min). We used 600 ml reactor for Fe and cation vacancies at both M1 and M2 positions have been isolated, demonstrating a whole spectrum of elec- Table 1. Synthesis conditions of the Li1-xFe1+x(PO4)1- trochemical performance (a competition of solid solution y(OH)4y samples. vs two phase (de)intercalation mechanism, different elec- Sample # Solution con- Hydrothermal Post- 21-27 trochemical potential, capacity and rate capability). No centration, treatment annealing wonder that great efforts were devoted to control the de- mol/l fect concentration in LiFePO4 by properly selecting the LiFePO4 synthesis and processing conditions. #1 1.7*10-3 Heating up to No o Until now the polyanion part of the LiFePO4 structure #2 3.4*10-3 210 C for 45 No has been entirely excluded from contributing to LiFePO4 min, followed by defect chemistry.28 Indeed, the prohibitively high for- immediate fast mation energy of 3.56 eV for the V''' vacancies puts their cooling PO4 expected concentration below detectable limit.29 Never- #3 0.1 Heating up at No o theless, there are experimental observations demonstrat- #4 0.2 190 C for 3.5 h, No followed by ing some phosphorus deficiency in LiFePO4. Amisse et al. #5 0.35 No refined the 8% P5+ vacancy concentration from powder spontaneous No neutron diffraction data in a highly defective triphylite #6 0.65 cooling. 3+ 2+ 3+ [Li0.85Fe 0.15]M1[Fe 0.71Fe 0.19Li0.09]M2P0.92O4 prepared by a #7 0.1 Yes 23 low-temperature co-precipitation method. This appar- #8 0.65 Yes ent yet unexplained lack of phosphorus looks particularly astonishing, as extracting the highly charged P5+ cation #9 0.907 Heating up to Yes 200 oC for 2h, from a PO tetrahedron leaves four strongly undercoordi- 4 followed by an- nated oxygen atoms and must be energetically highly un- nealing for 1 h favorable. In this contribution, we provide a plausible and spontaneous explanation of this phenomenon and demonstrate that 3- cooling. PO4 can be partially replaced by the hydroxyl groups in the course of low-temperature hydrothermal synthesis of these synthesis in order to increase reaction yield. G3 transmission electron microscope equipped with the The “reference” sample #9 was obtained via slightly dif- probe aberration corrector and operated at 200 kV. Ener- ferent route enabling synthesis of high-performance oli- gy dispersive X-ray (EDX) analysis was performed with an vine-type phosphate materials.
Details
-
File Typepdf
-
Upload Time-
-
Content LanguagesEnglish
-
Upload UserAnonymous/Not logged-in
-
File Pages14 Page
-
File Size-