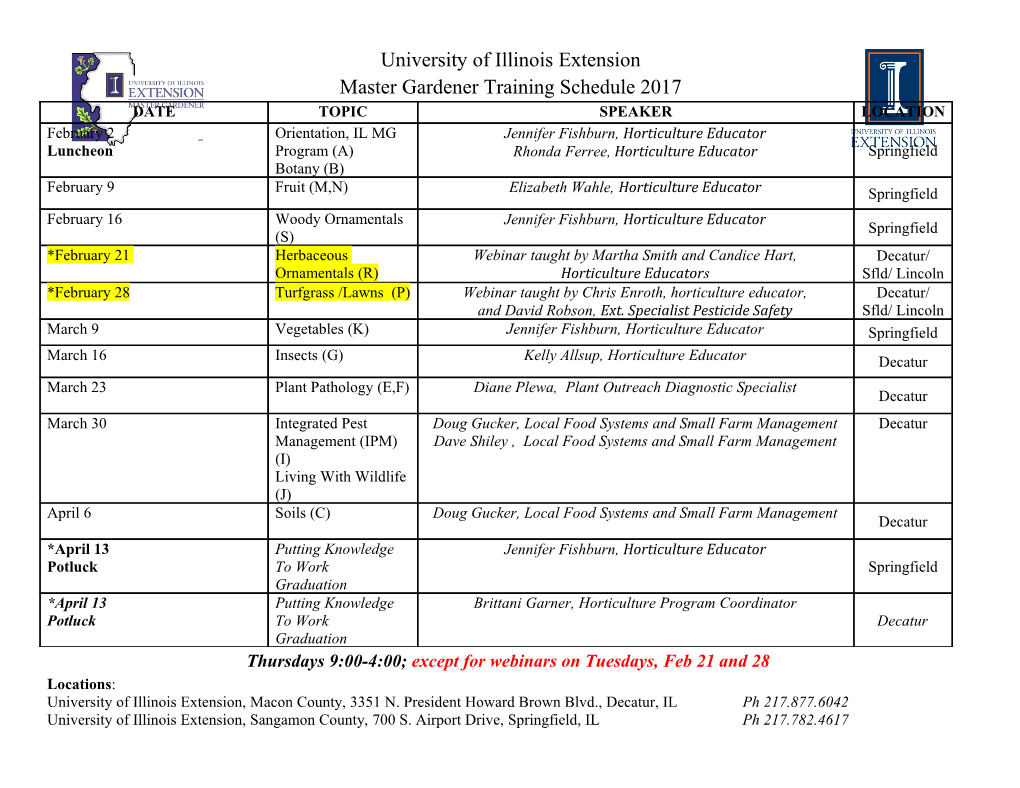
AMERICAN METEOROLOGICAL SOCIETY Monthly Weather Review EARLY ONLINE RELEASE This is a preliminary PDF of the author-produced manuscript that has been peer-reviewed and accepted for publication. Since it is being posted so soon after acceptance, it has not yet been copyedited, formatted, or processed by AMS Publications. This preliminary version of the manuscript may be downloaded, distributed, and cited, but please be aware that there will be visual differences and possibly some content differences between this version and the final published version. The DOI for this manuscript is doi: 10.1175/MWR-D-17-0329.1 The final published version of this manuscript will replace the preliminary version at the above DOI once it is available. If you would like to cite this EOR in a separate work, please use the following full citation: Keller, J., C. Grams, M. Riemer, H. Archambault, L. Bosart, J. Doyle, J. Evans, T. Galarneau, K. Griffin, P. Harr, N. Kitabatake, R. McTaggart-Cowan, F. Pantillon, J. Quinting, C. Reynolds, E. Ritchie, R. Torn, and F. Zhang, 2018: The Extratropical Transition of Tropical Cyclones Part II: Interaction with the midlatitude flow, downstream impacts, and implications for predictability. Mon. Wea. Rev. doi:10.1175/MWR-D-17-0329.1, in press. © 2018 American Meteorological Society Manuscript (non-LaTeX) Click here to access/download;Manuscript (non-LaTeX);ET Review II - revision 2- for submission.docx 1 2 3 The Extratropical Transition of Tropical Cyclones Part II: Interaction with the 4 midlatitude flow, downstream impacts, and implications for predictability 5 6 Julia H. Keller1 7 World Meteorological Organization, Geneva, Switzerland 8 Christian M. Grams 9 Institute for Atmospheric and Climate Science, ETH Zürich, Zürich, Switzerland and Institute of 10 Meteorology and Climate Research (IMK-TRO), Karlsruhe Institute of Technology, Karlsruhe, 11 Germany 12 Michael Riemer 13 Johannes Gutenberg-Universität Mainz, Mainz, Germany 14 Heather M. Archambault 15 NOAA/Geophysical Fluid Dynamics Laboratory, Princeton, New Jersey 16 Lance Bosart 17 Department of Atmospheric and Environmental Sciences, University at 18 Albany, State University of New York, Albany, New York 19 James D. Doyle 20 Naval Research Laboratory, Monterey, California 21 Jenni L. Evans 22 The Pennsylvania State University, University Park, Pennsylvania 23 Thomas J. Galarneau Jr. 24 The University of Arizona, Tucson, Arizona 25 Kyle Griffin 26 RiskPulse, Madison, Wisconsin 27 28 Corresponding Author: Julia H. Keller, World Meteorological Organization, Geneva, Switzerland, 29 email: [email protected] 1 Former affiliation: Deutscher Wetterdienst, Research & Development, Numerical Models, Offenbach, Germany 1 30 Patrick A. Harr 31 Naval Postgraduate School, Monterey, California 32 Naoko Kitabatake 33 Meteorological College, Kashiwa, Chiba, Japan 34 Ron McTaggart-Cowan 35 Numerical Weather Prediction Research Section, Environment and Climate Change Canada 36 Florian Pantillon 37 Institute of Meteorology and Climate Research (IMK-TRO), Karlsruhe Institute of Technology, 38 Karlsruhe, Germany 39 Julian Quinting2 40 School of Earth, Atmosphere and Environment, and ARC Centre of Excellence for Climate System 41 Science, Monash University, Clayton, Australia and Institute of Meteorology and Climate Research 42 (IMK-TRO), Karlsruhe Institute of Technology, Karlsruhe, Germany 43 Carolyn A. Reynolds 44 Naval Research Laboratory, Monterey, California 45 Elizabeth A. Ritchie 46 University of New South Wales, Canberra, Australia 47 Ryan D. Torn 48 Department of Atmospheric and Environmental Sciences, University at 49 Albany, State University of New York, Albany, New York 50 Fuqing Zhang 51 The Pennsylvania State University, University Park, Pennsylvania 2 52 Abstract 53 The extratropical transition (ET) of tropical cyclones often has an important impact on 54 the nature and predictability of the midlatitude flow. This review synthesizes the current 55 understanding of the dynamical and physical processes that govern this impact and highlights 56 the relationship of downstream development during ET to high-impact weather, with a focus 57 on downstream regions. It updates a previous review from 2003 and identifies new and 58 emerging challenges, and future research needs. First, the mechanisms through which the 59 transitioning cyclone impacts the midlatitude flow in its immediate vicinity is discussed. This 60 ‘direct impact’ manifests in the formation of a jet streak and the amplification of a ridge 61 directly downstream of the cyclone. This initial flow modification triggers or amplifies a 62 midlatitude Rossby wave packet, which disperses the impact of ET into downstream regions 63 (‘downstream impact’) and may contribute to the formation of high-impact weather. Details 64 are provided concerning the impact of ET on forecast uncertainty in downstream regions and 65 on the impact of observations on forecast skill. The sources and characteristics of the 66 following key features and processes that may determine the manifestation of the impact of 67 ET on the midlatitude flow are discussed: the upper-tropospheric divergent outflow, mainly 68 associated with latent heat release in the troposphere below, and the phasing between the 69 transitioning cyclone and the midlatitude wave pattern. Improving the representation of 70 diabatic processes during ET in models, and a climatological assessment of the ET’s impact 71 on downstream high-impact weather are examples for future research directions. 72 73 1 74 1. Introduction and motivation 75 Tropical cyclones (TC) that move poleward often interact with the midlatitude flow, 76 undergo profound structural changes, and transition into extratropical cyclones. This process 77 is known as extratropical transition (ET; Sekioka 1956; Palmén 1958). In recent years, 78 several ET cases were associated with extreme weather events, thus attracting the attention of 79 the general public. Hurricane Sandy (2012) inflicted widespread damage and severe 80 disruption of public life along the Northeast U.S. coast as it underwent ET (Blake et al. 2013; 81 Halverson and Rabenhorst 2013). Hurricane Gonzalo (2014), having undergone ET, tracked 82 across Europe and brought flooding and extreme winds to the Balkans (Brown 2015; Feser et 83 al. 2015). Extreme precipitation associated with Tropical Storm Etau (2015) during and after 84 its ET over Japan flooded areas north and east of Tokyo (AIR Worldwide 2015; Kitabatake et 85 al. 2017). In these examples, the high-impact weather was associated directly with the 86 transitioning cyclone. Such impacts, along with the structural evolution of the cyclone during 87 ET, are discussed in the first part of this review (Evans et al. 2017; referred to as Part I in the 88 remainder of this manuscript). 89 Extratropical transition may also lead to high-impact weather far downstream from 90 the actual cyclone. A prominent example for such a ‘downstream impact’ is provided by the 91 ET of Supertyphoon Nuri (2014) in the western North Pacific, displayed in Fig. 1. At the 92 onset of ET, Nuri moves poleward and starts to interact with the midlatitude flow (Fig. 1a). 93 This results in the formation of a jet streak (Fig. 1b) and a poleward deflection of the jet near 94 the transitioning cyclone in conjunction with the development of a ridge–trough couplet (Fig. 95 1b). At the same time a region of enhanced moisture flux—a so-called atmospheric river 96 (Zhu and Newell 1998)—forms ahead of the downstream trough. The ridge–trough couplet 97 continues to amplify, a new cyclone develops farther downstream, and the next downstream 98 ridge builds, which signifies the downstream propagation that arises from the initial local 2 99 changes in the jet near the site of ET (Fig. 1c). Meanwhile, Nuri reintensifies into a strong 100 extratropical cyclone and initiates cyclonic wave breaking over the western North Pacific 101 (Fig. 1c). Subsequently, the upper-level wave pattern amplifies further downstream, 102 establishing a high-amplitude ridge–trough couplet over North America. A heat wave 103 develops in the high-pressure conditions along the North American west coast, with highest 104 values occurring along the coast of California and over Alaska. The atmospheric river in the 105 western flank of the second downstream ridge (Fig. 1c) makes landfall in Alaska and British 106 Columbia, resulting in heavy precipitation (Fig. 1d). A cold-air outbreak occurs over the 107 continental and eastern North America. Further amplification of this pattern eventually leads 108 to a massive omega block over the west coast of North America and associated cold surges 109 and heavy snowfall in the continental and eastern U.S. (Bosart et al. 2015). Nuri is just one 110 example of the type of midlatitude flow modification due to ET. The processes acting during 111 such a midlatitude flow modification and the associated implications on downstream 112 extratropical regions are the subjects of this review. 113 Together with Part I, this review describes developments in our understanding of ET 114 since the first ET review by Jones et al. (2003). The review by Jones et al. (2003), referred to 115 as J2003 in the remainder of the manuscript, was motivated by the challenges that ET 116 typically poses to forecasters in terms of predicting the structural evolution of the 117 transitioning cyclone itself, and the high-impact weather that might be associated with it, 118 mostly in the immediate proximity of the storm. Since the publication of J2003 it has become 119 increasingly apparent that a forecasting challenge is also present for the region downstream 120 of ET because ET often leads to a basin-wide or even hemispheric reduction in the forecast 121 skill of numerical weather prediction (NWP) models. J2003 reviewed the then current 122 insights into ET and highlighted the need for a better understanding of the physical and 123 dynamic processes involved in ET, and their representation in NWP models. Since then, the 3 124 research community's understanding of the interactions that occur between a transitioning 125 cyclone and the midlatitude flow during ET has progressed considerably.
Details
-
File Typepdf
-
Upload Time-
-
Content LanguagesEnglish
-
Upload UserAnonymous/Not logged-in
-
File Pages82 Page
-
File Size-