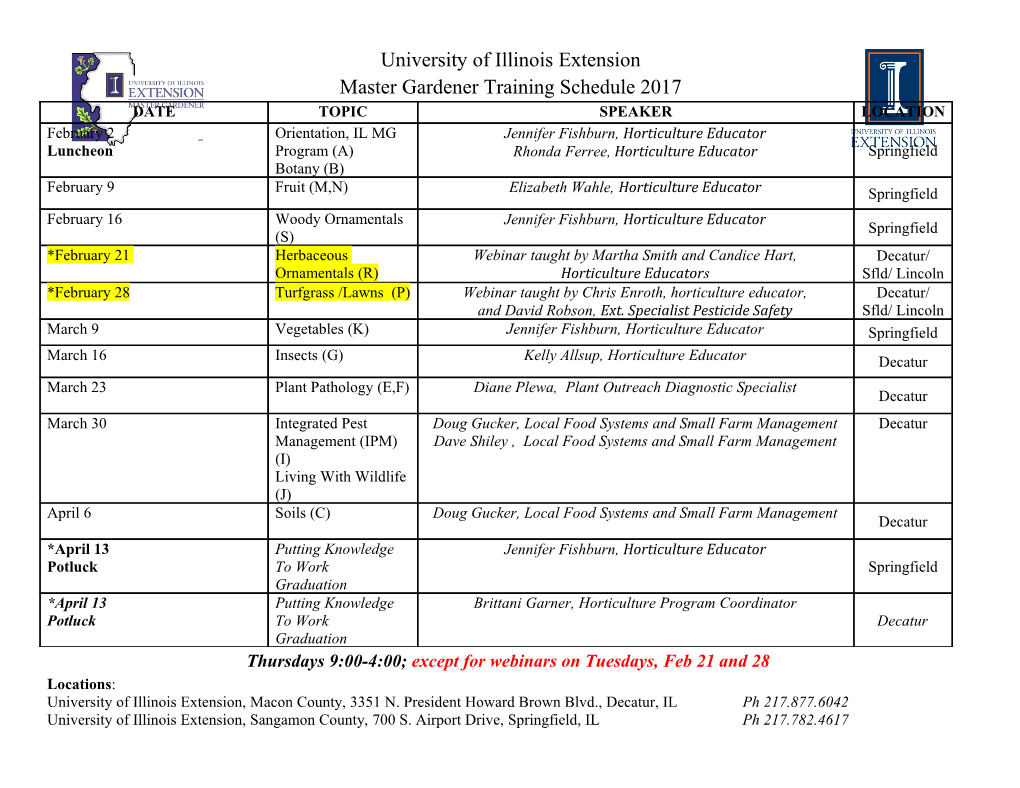
The fossil record of insect color illuminated by maturation experiments Maria E. McNamara1,2,*, Derek E.G. Briggs1,3, Patrick J. Orr2, Neal S. Gupta4, Emma R. Locatelli1, Lin Qiu1, Hong Yang4, Zhengrong Wang1, Heeso Noh5, and Hui Cao5 1Department of Geology & Geophysics, Yale University, New Haven, Connecticut 06520, USA 2UCD School of Geological Sciences, University College Dublin, Belfi eld, Dublin 4, Ireland 3Yale Peabody Museum of Natural History, Yale University, New Haven, Connecticut 06520, USA 4Laboratory for Terrestrial Environments, Department of Science and Technology, Bryant University, Smithfi eld, Rhode Island 02917, USA 5Department of Applied Physics, Yale University, New Haven, Connecticut 06520, USA ABSTRACT Pachyrrhynchus reticulatus (Coleoptera: Curcu- Structural coloration underpins communication strategies in many extant insects but its lionidae) (Fig. 4; Fig. DR5); the color-producing evolution is poorly understood. This stems, in part, from limited data on how color alters dur- nanostructure is a multilayer refl ector (Noyes et ing fossilization. We resolve this by using elevated pressures and temperatures to simulate the al., 2007) and a 3-D photonic crystal (V. Sara- effects of burial on structurally colored cuticles of modern beetles. Our experiments show that nathan, 2012, personal commun.), respectively. the color generated by multilayer refl ectors changes due to alteration of the refractive index The cuticle of C. raja is brightly refl ective and and periodicity of the cuticle layers. Three-dimensional photonic crystals are equally resistant iridescent; the elytra are predominantly green λ ≈ to degradation and thus their absence in fossil insects is not a function of limited preservation (peak wavelength [ max] 550 nm) with longi- potential but implies that these color-producing nanostructures evolved recently. Structural tudinal dorso-lateral red-orange bands (Fig. 1A, colors alter directly to black above a threshold temperature in experiments, identifying burial inset). The multilayer refl ector is located in the temperature as the primary control on their preservation in fossils. Color-producing nano- epicuticle (Fig. 1F). The cuticle of P. reticula- structures can, however, survive in experimentally treated and fossil cuticles that now are tus is black with yellow-orange iridescent bands black. An extensive cryptic record is thus available in fossil insects to illuminate the evolution (Figs. 4A and 4B) that comprise assemblies of of structural color. scales; each scale exhibits many microdomains that differ in color (Fig. 4B). This is due to INTRODUCTION 2012a). All biophotonic nanostructures in fossil varying orientations of photonic crystals with a The integumentary colors of extant organ- insects are multilayer refl ectors (McNamara et single-network diamond (Fd3m) nanostructure isms are generated by pigments or biophotonic al., 2011, 2012a); 3-D photonic crystals occur within the scale lumen (Fig. 4E). nanostructures that scatter incident light via in many groups of extant insects (e.g., Sarana- Specimens for decay were placed in individ- ordered or quasi-ordered variations in refractive than et al., 2010; Colomer et al., 2012; Wilts et ual glass jars with 200 ml natural lake water and index (Vukusic and Sambles, 2003). The latter al., 2012) but have not been recorded in fossils. 5 ml sediment (Dart Lake, Herkimer County, generate highly conspicuous visual signals used Colored fossil insects tend to be either abundant New York, USA) and degraded in the dark at 25 in species and sex recognition, mate choice, or absent in any individual fossil biota (McNa- ± 0.3 °C and 50 ± 0.5% humidity for 18 mo. and predator avoidance (Doucet and Meadows, mara et al., 2012b), suggesting the presence of Fragments of fresh and decayed elytron for 2009); they are the focus of intense research due a taphonomic “on/off” switch. It is not clear to maturation were wrapped in aluminum foil and to their biomimetic potential and the insights what extent these patterns in the fossil record of inserted into an autoclave pressurized using Ar they offer into animal behavior (Doucet and structural color refl ect real biological signals or gas at: 200 °C, 1 bar; 200 °C, 117 bar; 200 °C, Meadows, 2009). The gamut of structural color- artifacts induced during decay or burial. Here 250 bar; 200 °C, 500 bar; 270 °C, 500 bar; ation is especially diverse in insects (Kinoshita we use a novel experimental approach that inte- and 25 °C, 500 bar, each for 24 h. One-hour and Yoshioka, 2005) where striking visual grates high pressure–high temperature autoclave experiments were run at 200 °C, 250 bar, and effects are generated by multilayer refl ectors experiments with decay experiments to reveal at 250 °C, 250 bar. Conditions fl uctuated within and three-dimensional (3-D) photonic crys- the effects of decay, pressure, and temperature ±3 °C and ±0.35 bar. tals that produce phenomena such as metallic, on different biophotonic nanostructures. Our Samples of elytron were prepared for electron polarized, and UV refl ection, iridescence, and/ results reveal the controls on structural color microscopy as in McNamara et al. (2012a) and or opalescence (Doucet and Meadows, 2009; preservation in insects, and confi rm the poten- examined using a FEI XL-30 fi eld emission gun Parker, 2005). Indirect evidence from phyloge- tial of fossils to illuminate the evolutionary his- (FEG) environmental scanning electron micro- netic data indicates that insect structural color tory of insect color. scope (ESEM) at an accelerating voltage of has multiple independent origins driven primar- 15kV, and a Zeiss EM900 transmission electron ily by sexual selection, and possibly linked to METHODS microscope (TEM) at 80kV with an objective diurnality, color vision, and certain light envi- Beetles are the most abundant structurally aperture of 90 μm diameter. ronments (Doucet and Meadows, 2009; Seago colored fossil insects (Doucet and Meadows, Refl ectance spectra were obtained from ely- et al., 2009), but these models require testing. 2009). Our study therefore focused on the extant tra using the method described in McNamara Fossils are the only direct source of evidence leaf beetle Chrysochroa raja (Coleoptera: et al. (2012a). Predicted refl ection spectra were for the evolution of color, but the original col- Chry somelidae) (Figs. 1–3; Figs. DR1–DR4 obtained by analyzing variation in the refractive ors in Cenozoic insects have altered in ways in the GSA Data Repository1) and the weevil index of biophotonic nanostructures using two- that are not fully resolved (McNamara et al., dimensional Fourier analysis of digital TEM 1GSA Data Repository item 2013127, Figures micrographs (Prum and Torres, 2003).Values DR1–DR6 and Tables DR1–DR3, is available online *Current address: School of Earth Sciences, Uni- at www.geosociety.org/pubs/ft2013.htm, or on request for the high- and low-refractive index layers in versity of Bristol, Bristol BS8 1RJ, UK; E-mail: from [email protected] or Documents Secre- the multilayer refl ector of the cuticle of C. raja [email protected]. tary, GSA, P.O. Box 9140, Boulder, CO 80301, USA. are 1.68 and 1.55 (Noyes et al., 2007). GEOLOGY, April 2013; v. 41; no. 4; p. 487–490; Data Repository item 2013127 | doi:10.1130/G33836.1 | Published online 20 February 2013 GEOLOGY© 2013 Geological | April Society 2013 | ofwww.gsapubs.org America. For permission to copy, contact Copyright Permissions, GSA, or [email protected]. 487 Figure 1. Experimentally induced color change in Chryso chroa raja cu- ticles. A–E: Light micro- graphs of the cuticle surface of untreated specimens (A) and speci- mens treated to 200 °C, 117 bar (B); 200 °C, 250 bar (C); 200 °C, 500 bar (D); and 270 °C, 500 bar (E). F–J: Transmission electron micrographs of the epicuticular multi- layer refl ector for sam- ples in A–E. High- and low-refractive index layers exhibit high and low electron contrast, respectively. K–O: Ex- perimentally measured (observed) and predicted (using Fourier analysis) refl ectance spectra for cuticles in A–E. Cuticles for gas chromatography–mass spec- to 150 °C (10 °C min–1) and then to 315 °C (6 °C undecayed) cuticles heated to 200 °C and trometry (GC-MS) were stored at −80 °C for 24 min–1; held 20 min). treated to pressures of 117 bar, 250 bar, and λ h, crushed, and extracted in 2:1 CH2CL2:CH3OH. 500 bar for 24 h altered from green ( max = 554 λ λ The total lipid extract was analyzed by an Agi- RESULTS nm) to cyan ( max = 519 nm), blue ( max = 485 λ lent 7890A gas chromatograph interfaced to a The hue generated by a biophotonic nano- nm), and indigo ( max = 453 nm), respectively 5975C mass spectrometer. Gas chromatography structure is determined by both the periodicity (Figs. 1A–1D). Coloration disappeared during was performed at 70eV using an HP-1MS 60 m and refractive index of its constituent materials the 270 °C, 500 bar treatment (Fig. 1E). Pres- capillary column with 320 mm diameter and (Schultz and Rankin, 1985); any color change sure and peak wavelength are positively corre- 0.25 mm fi lm thickness, and He as carrier gas; observed in our experiments thus refl ects lated (Fig. DR2). Similar results in experiments concentrations of lipid compounds were evalu- alteration of one, or both, of these. The hue using degraded cuticles (Figs. DR1C–DR1G) ated using a 7683B Series autosampler. The GC of C. raja cuticles was unaltered after 18 mo. confi rm that decay processes have no discern- oven was programmed from 40 °C (held 1 min) decay (Figs. DR1A and DR1B). Fresh (i.e., able effect on the physical properties of the multilayer refl ector or the resultant hue. During pressure-temperature treatment, the decrease in wavelength is accompanied by a decrease in A 9,12,15 octanetrienoic linolenic acid acid the periodicity of the multilayer refl ector from methyl ester 1 161 nm to 128 nm (Figs.
Details
-
File Typepdf
-
Upload Time-
-
Content LanguagesEnglish
-
Upload UserAnonymous/Not logged-in
-
File Pages4 Page
-
File Size-