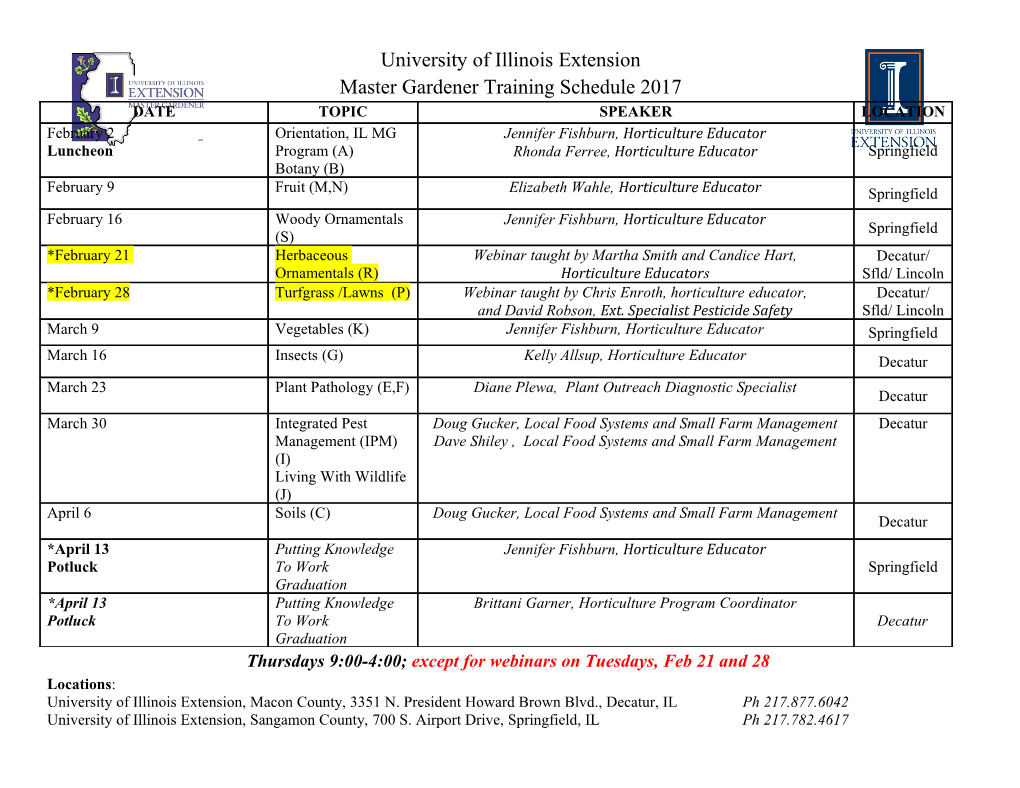
CALIFORNIA STATE UNIVERSITY, NORTHRIDGE SPACE COMMUNICATION THEORY AND PRACTICE A graduate project submitted in partial satisfaction of the requirements for the degree of the Masterr of Science 1n Engineering by Kristine Patricia Maine January, 1983 The project of Kristine Patricia Maine is approved: CDr. Ste~en A. Gad~ski) (Dr. ~y H. Pettit, Chair) California State University, Northridge ii ACKNOWLEDGEMENTS A deep sense of gratitude is expressed to my academic advisor, Dr. Ray Pettit, for his invaluable counsel and advisement during the course of this study. In addition, I express thanks to The Aerospace Corporation for the use of their facilities and personnel for the completion of this document. iii TABLE OF CONTENTS Title Page Acknowledgement iii Table of Figures v List of Tables vii List of Symbols viii Abstract xi I. Introduction 1 II. Space Communication System Components 2 III. Theory of Link Analysis 8 IV. Applications of Link Analysis 21 v. Summary 41 Appendix A--Signal Losses 45 Appendix B--Space Shuttle Design Model 54 Appendix C--Communication Satellite Systems 62 Footnotes 67 Bi biliography 70 iv TABLE OF FIGURES Figure Number Title 1 Simplified Transponder Block Diagram 3 Showing a Single Channel Transponder 2 TWT Characteristics 4 3 Typical Antenna Beamwidth Pattern 7 4 Satellite Communication System 9 5 Uplink Model 10 6 Downlink Model 13 7 Satellite Communication Transmitter­ 16 to-Receiver with Typical Loss and Noise Sources 8 Zenith Attenuation Values Expected to 18 be Exceeded 2% of the Year at Mid­ latitudes 9 Probability of Error for Binary Digital 20 Modulation Schemes 10 Jupiter Atmospheric Loss for a 10 Bar 32 Pressure Level 11 Link Margin as a Function of Periapsis 35 Distance 12 Bit Error Probability vs Energy-per­ 42 Bit to Noise Density Ratio 13 Typical AM/PM Conversion Phase Shift 47 14 Model of AM/PM Nonlinearities in a 48 TWT Amplifier 15 CW Phase-Locked Loop 55 16 Costas Loop 56 17 Equivalent Baseband Phase Error Model 57 of Shuttle Phase-Locked Receiver v 18 Estimated Phase Noise Loop Density 61 19 Evolution of INTELSAT Space System 63 vi LIST OF TABLES Table Number Title 1 High Definition Television 23 Satellite Broadcasting 2 DBS Satellite Link Analysis 25 3 Satellite-to-Satellite Link 27 4 Terminal Performance Characteristics­ 29 Shipboard Terminal 5 Terminal Performance Characteristics­ 29 COMSAT Labs Terminal 6 Ship-to-Satellite-to-Earth Link Budget 30 Calculation 7 Jupiter Probe Communication System 36 Parameters 8 Hypothetical Military Communication 38 Uplink Calculation vii LIST OF SYMBOLS saturated flux density P* outpower corresponding to the saturated flux density s RF transmitter power input backoff value output backoff value G power gain transmitter antenna gain satellite antenna gain (uplink) G receiver gain r satellite gain (downlink) uplink wavelength wavelength downlink wavelength antenna aperature efficiency physical area of antenna A effective area of satellite antenna e R uplink distance u downlink distance range L additional uplink losses u additional downlink losses viii L space loss s transmitting polarization and filter losses 1 tpf receiving polarization and filter losses L rpf N noise density 0 23 k Boltzmann's constant, 1.38 x lo- J/°K system effective temperature T s T antenna temperature a T receiver temperature r NF noise figure C/N carrier-to-noise density ratio 0 C/N carrier-to-noise power ratio B receiver bandwidth bit rate bit-energy-to-noise density ratio bit-energy-to-jammer noise density ratio error probability M link margin J/S jammer power-to-signal power ratio w transmission bandwidth PG processing gain low frequency frequency high frequency constant for flicker noise white noise component flicker noise frequency A(t) input envelope ix 8(t) phase noise variation G(t) VCO phase estimation phase noise fluctuation receiver phase noise input signal amplitude n ( t) white gaussian noise g cp phase error F(p) loop filter transfer function K open loop gain probability density function mean squared value of phase error X ABSTRACT SPACE COMMUNICATION THEORY AND PRACTICE by Kristine Patricia Maine Master of Science in Engineering Link power budgets for estimating the quality of signal transmission have been developed for space communication. Essentially, a link power budget can be set up, a balance sheet of gains and losses for the signal transmission path, as a tool to analyze the performance of the entire communication link. Examples in this paper illustrate the uses of link power budget analysis for geostationary communication satellites, deep space orbiting probes, satellite-to-satellite crosslinks, and ship-satellite-shore communication links. xi SPACE COMMUNICATION THEORY AND PRACTICE I. Introduction Present-day space communications utilize microwave engineering, a distinguished technology, that has greatly advanced since World War II. During this same period, the developement of statistical communications provided the means for understanding the fundamental relationships of transmission bandwidth, carrier-to-noise ratio, and attenuation. The development of communication link performance analysis based on statistical communication theory has been applied to space communications. Essentially, signal power, antenna gain, noise, and signal loss are analyzed over the signal transmission path. The signal transmission path starts at an earth ground station which transmits a signal through space. A satellite or spacecraft receives the signal and transmits it to another earth station in the network. A "link power budget" is commonly set up as a balance sheet of gains and losses for the transmission path and is used to analyze the performance of the entire communication link. Link analysis is primarily used to predict link performance by a carrier-to-noise density ratio, a value indicating the strength of the signal over the 1 2 ' . noise encountered along its path. In order to understand the parameters used in a link analysis, a brief description of components found in space communication systems is given in the next section. II. Space Communication System Components Most communication satellites contain several (four or more) parallel transponders with several narrow beam antennas for different classes of users (mobile terminals or fixed ground stations). Figure 1 illustrates a model of a single channel transponder. Multiple input sinusoidal waves which carry digital information enter the transponder in frequency band fu and exit in band fd. The frequency bands are sufficiently separated to prevent "ring-around" oscillation in the transponder. Ring-around oscillation is a term used to describe the effect of fu being indistinguishable from fd implying fd will be included with the other uplink signals. A bandpass filter allows the f frequency band to enter the transponder and excludes any other u frequency. Following the filter, a preamplifier strengthens the signal which travels into a frequency converter changing f to u fd. The traveling wave tube (TWT), a power amplifier, strengthens fd significantly so that it may be detected at a ground station. Most TWTs utilize vacuum-tube technology with nonlinear power characteristics, as shown in Figure 2. The flux density necessary to saturate the TWT is denoted by Q* and corresponding output power u is P* • Frequently, the satellite is operated backed off from s saturation mode to avoid nonlinear distortions. The input and output MULTIPLE INPUT SIGNALS f \t/ u BANDPASS BANDPASS FILTER r ___ -, AMPLIFIER LOW NOISE 1 X I PREAMP I LIMITER I I r--__..___ _, TRAVELING I 1 LOCAL I WAVE TUBE I OSCILLATOR 1 t_: ______ ~ INPUT OUTPUT FREQUENCY CHANNEL CHANNEL CONVERTER ~ ~ lllf fu fd Figure 1. Simplified Transponder Block Diagram Showing a Single Channel Transponder.l w --BOI 20 10 0 0 BOo -::-0..Vl 22. 0 SINGLE CARRIER~ s ;g 12.0 10 l 0 r-­ r--4 lo.... Q) 2.0 20 > 0 0.. 0::: LLJ -8.0 -_J SINGLE CARRIER <( CQ SATURATION 0 _J (.!) - 113. 7 - 103. 7 - 93. 7 - 83. 7 - 73. 7 2 dBW I m AT MINIMUM ATTENUATOR SETTING, .Q:~ 2 Figure 2. TWT Characteristics +:'- "' 5 backoff values are respectively denoted as B0 and B0 • 1 0 Satellite transponders are often multichannel in configuration. The transponder is channelized by a frequency-selector filter to allow different frequency bands to be h~ndled by separate amplifiers and antennas. Transponder channelization is usually applied in order to increase the total downlink power by using parallel power amplifiers (TWTs) inside the satellite. The number of signals handled by a single TWT can then be decreased, reducing the nonlinear effects. However, one of the most important features of the transponder is its ability to isolate signals from small mobile terminals and large fixed 3 ground stations by using separate channels inside one transponder. The multiple sinusoidal waves that may appear at the TWT input can cause significant intermodulation products in the output channel bandwidth unless the power is backed off along the power curve shown in Figure 2. Intermodulation products refer to the nonlinear effects created by a cross-product of two or more input signals. Because the same antenna can be used for transmitting and receiving, the TWT must be heavily filtered to prevent cross-products from falling in the receiver frequency band and saturating the preamplifier. The antenna of a ground station or a satellite acts as a transducer between free-space propagation and the guided-wave propagation. A measure of the ability of an antenna to concentrate energy in a particular direction is called "gain". Power gain u defined as G = maximum radiation intensity from subject antenna (1) radiation intensity from (lossless) isotropic source with same power input. 6 The relationship between the gain and the beamwidth of an antenna depends on the distribution of the area across the aperture. A typical beamwidth pattern in polar coordinates for a parabolic antenna (the most common type of antenna used in space communications) is illustrated in Figure 3.
Details
-
File Typepdf
-
Upload Time-
-
Content LanguagesEnglish
-
Upload UserAnonymous/Not logged-in
-
File Pages79 Page
-
File Size-