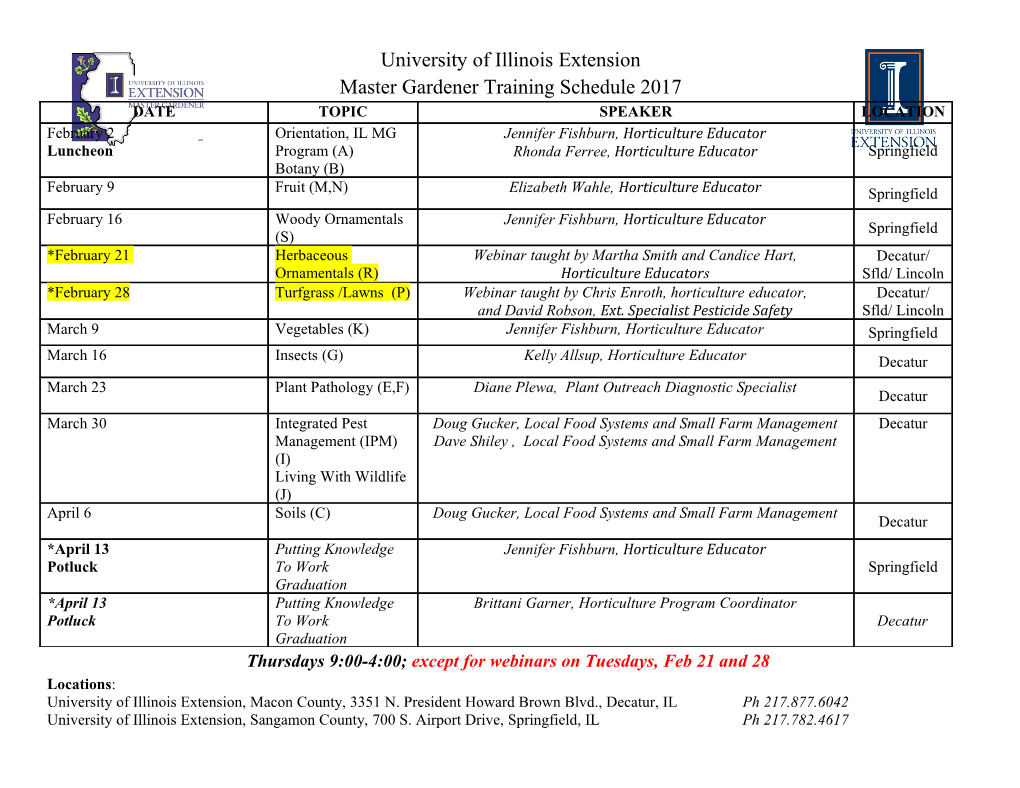
Published OnlineFirst September 24, 2014; DOI: 10.1158/2159-8290.CD-14-0729 reseArch Article Mutation-Specific RAS Oncogenicity Explains NRAS Codon 61 Selection in Melanoma Christin E. Burd1,2, Wenjin Liu3,4, Minh V. Huynh5, Meriam A. Waqas1, James E. Gillahan1,2, Kelly S. Clark3,4, Kailing Fu3,4, Brit L. Martin1, William R. Jeck3,4,. George P Souroullas3,4, David B. Darr3,4, Daniel C. Zedek6, Michael J. Miley7, Bruce C. Baguley8, Sharon L. Campbell4,5, and Norman E. Sharpless3,4 Abstrt Ac NRAS mutation at codons 12, 13, or 61 is associated with transformation; yet, in melanoma, such alterations are nearly exclusive to codon 61. Here, we compared the melanoma susceptibility of an NrasQ61R knock-in allele to similarly designed KrasG12D and NrasG12D alleles. With concomitant p16INK4a inactivation, KrasG12D or NrasQ61R expression efficiently promoted melanoma in vivo, whereas NrasG12D did not. In addition, NrasQ61R mutation potently cooperated with Lkb1/Stk11 loss to induce highly metastatic disease. Functional comparisons of NrasQ61R and NrasG12D revealed little difference in the ability of these proteins to engage PI3K or RAF. Instead, NrasQ61R showed enhanced nucleotide binding, decreased intrinsic GTPase activity, and increased stability when compared with NrasG12D. This work identifies a faithful model of human NRAS-mutant melanoma, and suggests that the increased melanomagenecity of NrasQ61R over NrasG12D is due to heightened abundance of the active, GTP-bound form rather than differences in the engagement of downstream effector pathways. SIGNIFICANCE: This work explains the curious predominance in human melanoma of mutations of codon 61 of NRAS over other oncogenic NRAS mutations. Using conditional “knock-in” mouse models, we show that physiologic expression of NRASQ61R, but not NRASG12D, drives melanoma formation. Cancer Discov; 4(12); 1–10. ©2014 AACR. 1Department of Molecular Genetics, The Ohio State University, Columbus, Note: Supplementary data for this article are available at Cancer Discovery Ohio. 2Department of Molecular and Cellular Biochemistry, The Ohio State Uni- Online (http://cancerdiscovery.aacrjournals.org/). 3 versity, Columbus, Ohio. Department of Genetics, University of North Carolina Corresponding Author: Norman E. Sharpless, The Lineberger Compre- 4 School of Medicine, Chapel Hill, North Carolina. The Lineberger Comprehen- hensive Cancer Center, University of North Carolina School Medicine, CB sive Cancer Center, University of North Carolina School of Medicine, Chapel #7295, Chapel Hill, NC 27599-7295. Phone: 919-966-1185; Fax: 919- 5 Hill, North Carolina. Department of Biochemistry and Biophysics, University 966-8212; E-mail: [email protected] of North Carolina School of Medicine, Chapel Hill, North Carolina. 6Department of Dermatology, University of North Carolina School of Medicine, Chapel Hill, doi: 10.1158/2159-8290.CD-14-0729 North Carolina. 7Department of Pharmacology, University of North Carolina ©2014 American Association for Cancer Research. School of Medicine, Chapel Hill, North Carolina. 8Auckland Cancer Society Research Centre, University of Auckland, Auckland, New Zealand. OF1 | CANCER DISCOVERY December 2014 www.aacrjournals.org Downloaded from cancerdiscovery.aacrjournals.org on September 25, 2021. © 2014 American Association for Cancer Research. Published OnlineFirst September 24, 2014; DOI: 10.1158/2159-8290.CD-14-0729 iNtrODUctiON mediators of mutation-specific therapeutic response (14). Together, these results suggest that distinct, codon-specific One third of all human cancers harbor activating KRAS, properties of RAS mutations have important clinical and HRAS, or NRAS mutations, which localize predominantly to biologic implications. codons 12, 13, or 61 (1, 2). RAS proteins function as canonical Cancers display tissue-specific preferences for mutation of GTPase switches, binding to effectors in the presence of GTP the RAS homologs (Supplementary Table S1). In melanoma, and activating downstream signaling pathways to influence NRAS is by far the most frequently mutated RAS isoform, cellular proliferation, differentiation, and survival. The return and notably, 84% of these mutations localize to codon 61 of RAS to an inactive, GDP-bound state is catalyzed by GTPase- versus only 7% to glutamine 12 (Supplementary Table S1). A activating proteins (GAP), which stimulate the weak, intrinsic similar preference for codon 61 mutations is noted in thyroid GTPase activity of these proteins. Mutations at codons 12 or cancer, but is not observed in other cancer types. Codon 12 13 render RAS proteins insensitive to GAP activity, resulting and 13 mutations constitute more than 90% of KRAS muta- in constitutive, oncogenic signaling (3). Similarly, mutation of tions observed in human colon, pancreatic, lung, and ovarian Q61, a catalytic residue required for efficient GTP hydrolysis, cancers (Supplementary Table S1 and ref. 1). Likewise, glycine impedes the return of RAS to an inactive GDP-bound state (4). 12 is the most common site of NRAS mutation in acute mye- Historically, RAS proteins with codon 12, 13, or 61 altera- loid leukemia (Supplementary Table S1). The mechanistic tions have been considered oncogenic equivalents; however, basis for codon 61 selection in melanoma and thyroid cancer recent clinical observations suggest functional differences for is unclear. Some have suggested that cytosine to thymidine each RAS mutation. For example, in colorectal cancer, KRAS transversions caused by ultraviolet (UV) light may explain mutational status is used as a prognostic indicator of resist- the preference for certain mutations in melanoma, but the ance to therapy with EGFR antibodies (e.g., cetuximab; refs. majority of codon 61 mutations do not exhibit a characteris- 5–8). Retrospective analyses, however, of “all-comer” trials tic UV-damage signature (15). Alternatively, it is possible that suggest mutational specificity in this regard: patients harbor- codon mutation preferences reflect differences in oncogenic ing KRAS codon 13 mutations appear to benefit from cetuxi- signaling. mab therapy, whereas those with codon 12 mutations were Comparing the oncogenic potential of various RAS unresponsive (9–13). Moreover, progression-free survival on mutants is challenging for several reasons. RAS gene dosage targeted therapies may also be codon-specific in non–small clearly influences downstream signaling, and artifacts of RAS cell lung cancer (NSCLC; ref. 14). Here, molecular modeling overexpression are well described. Likewise, endomembrane and reverse-phase protein analysis pinpointed differential localization is critical for physiologic RAS signal transduc- effector engagement and downstream signaling as potential tion (16), and may not be adequately recapitulated using December 2014 CANCER DISCOVERY | OF2 Downloaded from cancerdiscovery.aacrjournals.org on September 25, 2021. © 2014 American Association for Cancer Research. Published OnlineFirst September 24, 2014; DOI: 10.1158/2159-8290.CD-14-0729 RESEARCH ARTICLE Burd et al. A G12D LSL-Nras Figure 1. Activation of LSL-NrasQ61R or LSL-NRASG12D (*G12D) slows melanocyte proliferation. A, diagrammatic representa- tion of the LSL-NrasG12D (17) and LSL-NrasQ61R alleles in the 0 PUROSA 3 STOP SD 1 2 3 4 5 6 ë presence or absence of CRE recombinase. Green triangles (*G12D) denote lox P sites and unnumbered black boxes represent 0 1 2 3 4 5 6 FRT sites. Blue boxes indicate the location of a puromy- + CRE cin (PURO) resistance cassette. SA, splice acceptor; SD, splice donor; 3× STOP, transcription and translational stop LSL-NrasQ61R sequence. B, PCR genotyping of melanocytes treated for 6 days with ethanol vehicle (E; lane 7) or 1.0 µmol/L 4-OHT (*Q61R) (lane 7). DNA from mice of the indicated genotypes is 0 SA 4ë STOP 1 2 3 4 5 6 included as a control (lanes 3–5). WT, wild-type. C, melano- cytes cultured as in B were incubated with EdU, a thymidine (*Q61R) analogue, for 16 hours and then analyzed by flow cytometry. EdU incorporation in vehicle-treated cells was set to 100%. + CRE 0 1 2 3 4 5 6 Each dot represents a biologic replicate with the mean and standard error of the mean indicated by black lines. Concen- trations of 4-OHT are provided in µmol/L. B O 2 MW H WT/WT LSL/WT LSL/LSL LSL/LSL LSL/LSL +CRE - WT G12D - LSL +CRE - WT Q61R - LSL 4-OHT: –– –– E 1.0 Lane: 1 2 3 4 5 6 7 C 120 100 80 60 12D/12D TpN 40 61R/61R TpN 20 No CRE Percentage of change in EdU 0 0 0.5 1.0 0 0.5 1.0 1.0 [4-OHT] exogenous protein expression. In addition, genetic alterations followed by a single missense mutation in the endogenous private to a given cell line or tumor sample could obscure Ras gene (G12D or Q61R, respectively; Fig. 1A). The codon distinct functions of individual RAS mutants. To circumvent 12 LSL-Kras and Nras alleles have been previously described these issues, we generated a knock-in allele (LSL-NrasQ61R) (17, 18). We generated and confirmed a related LSL-NrasQ61R allowing for the conditional, tissue-specific, and somatic allele using standard homologous recombination followed expression of NRASQ61R under control of the endogenous by Southern blot, PCR, and genomic sequencing (Supple- promoter. We used this allele in combination with similarly mentary Fig. S1A–S1D). To minimize strain-specific effects, designed knock-in NrasG12D (17) and KrasG12D (18) models to all alleles were backcrossed more than seven generations to compare the transforming potential of each mutant when C57BL/6J in the presence of a conditional p16INK4a knockout expressed at physiologic levels in melanocytes. allele (p16L; ref. 19) and a melanocyte-specific, 4-hydrox- ytamoxifen (4-OHT)–inducible CRE recombinase (Tyr-CRE- T2 resULTS ER ; ref. 20). Cohorts of all three alleles were born at normal Mendelian ratios (data not shown) and showed no defects in Generation and Characterization development or fertility. of LSL-Nras Alleles Using primary melanocytes derived from syngeneic Tyr-CRE- To compare the ability of NRAS mutants to promote ERT2 p16L/L LSL-NrasG12D/G12D (TpN12D/12D) or LSL-NrasQ61R/Q61R melanoma formation, we used three conditional knock-in (TpN61R/61R) neonates, we verified the functionality of the LSL alleles: LSL-NrasG12D (17), LSL-KrasG12D (18), and LSL-NrasQ61R.
Details
-
File Typepdf
-
Upload Time-
-
Content LanguagesEnglish
-
Upload UserAnonymous/Not logged-in
-
File Pages13 Page
-
File Size-