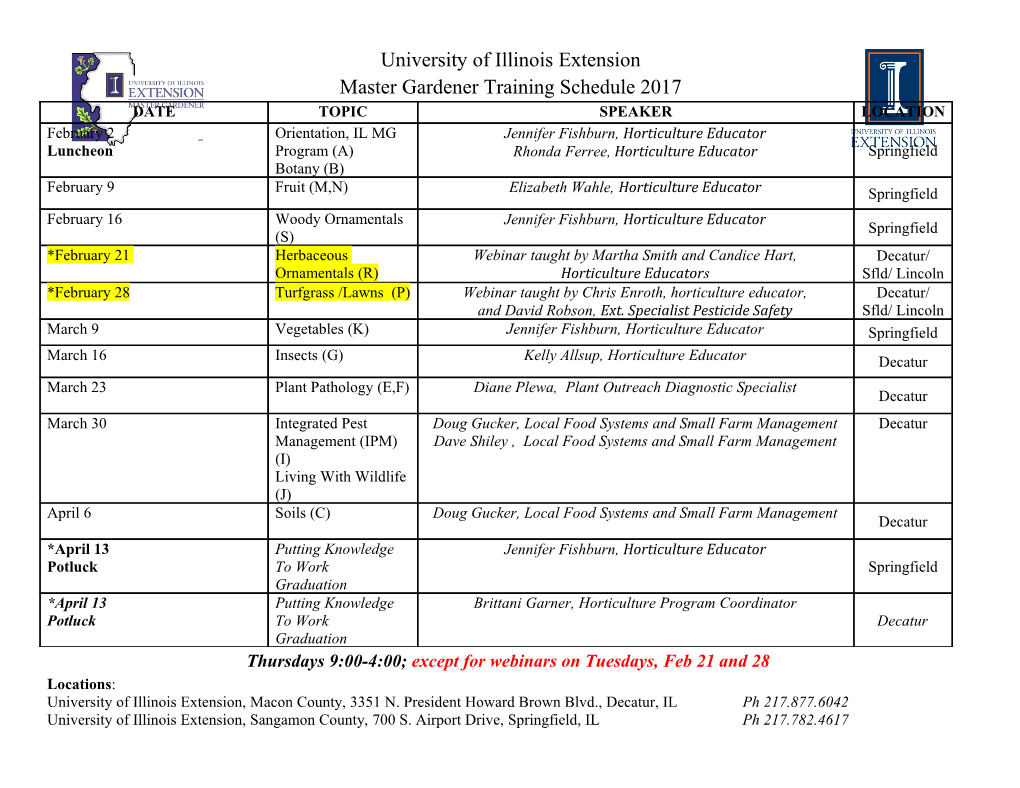
Energy, Environment and Sustainable Development 1998-2002 Call identifier: EESD-ENV-99-2 Key Action 2: Global Change, Climate and Biodiversity Area 2.4 European Component of the Global Observing Systems RTD priority-2.4.1: Better Exploitation of Existing Data and Adaptation of Existing Observing Systems Final report Quantification and Interpretation of Long-Term UV-Visible Observations of the Stratosphere Acronym: QUILT Project number: EVK2-2000-00545 Contract N°: EVK2-CT-2000-00059 Date of preparation: 29 November 2004 1 1 QUILT Final Report I.1QUILT Final Report I.1 QUILT - Final Report Project coordinator: Geir O. Braathen, Norwegian Institute for Air Research Report edited by: Kerstin Stebel, Norwegian Institute for Air Research Contributions from: Norwegian Institute for Air Research (NILU), Kjeller, Norway Geir O. Braathen, Kåre Edvardsen, Britt Ann K. Høiskar, Arve Kylling, Kerstin Stebel, and Kjersti K. Tørnkvist Belgian Institute for Space-Aeronomy (IASB-BIRA), Brussels, Belgium Caroline Fayt, François Hendrick, and Michel Van Roozendael Institute of Environmental Physics, University of Bremen (IUP-UHB), Bremen, Germany John P. Burrows, Sixten Fietkau, Thomas Medeke, Hilke Oetjen, Andreas Richter, Björn-Martin Sinnhuber, Mark Weber, and Folkard Wittrock Service d’Aéronomie du Centre National de la recherche Scientifique (CNRS-DR5- SA), Paris, France Florence Goutail, Aude Mieville, Franck Lefevre, Jean-Pierre Pommereau The Institute for Environmental Physics at the University of Heidelberg (U- Heidelberg), Heidelberg, Germany Andre Butz, Barbara Dix, Udo Frieß, Klaus Pfeilsticker, Ulrich Platt, Irene Pundt, Roman Sinreich, Christoph von Friedeburg, and Thomas Wagner University of Leeds (UNIVLEEDS), School of Environment, United Kingdom Martyn Chipperfield and Wuhu Feng NERC-British Antarctic Survey (BAS), Cambridge, United Kingdom Howard Roscoe and Laurent Denis National Institute of Aerospace Technology (INTA), Torrejón de Ardoz, Spain Manuel Gil, Monica Navarro, Olga Puentedura, and Margarita Yela National Institute for Space Research (SRON), Utrecht, The Netherlands Jos de Laat Institute of Atmospheric Sciences and Climate (CNR-ISAC), Bologna, Italy Daniele Bortoli, Giorgio Giovanelli, Ivan Kostadinov, Andrea Petritoli, and Fabrizio Ravegnani Solar-Terrestrial Influences Laboratory, Stara Zagora Department, Bulgarian Academy of Sciences (STIL-BAS) Rolf Werner QUILT Final Report I.2 Acronym List AMF Air Mass Factor CTM Chemical Transport Model DOAS Differential Optical Absorption Spectroscopy DSCD Differential Slant Column Density EASOE European Arctic Stratospheric Experiment EC European Commission ECMWF European Centre for Medium-Range Weather Forecasts ENVISAT Environmental Satellite ERS European Remote Sensing Satellite ESA European Space Agency EU European Union GATO Global Atmospheric Observations GB Ground-Based GCM General Circulation Model GOME Global Ozone Monitoring Experiment NDSC Network for the Detection of Stratospheric Change NRT Near-Real Time OA Off-axis DOAS ODP Ozone Depletion Potential ODS Ozone Depleting Substances PMT Project Management Team PSC Polar Stratospheric Cloud RTD Research, Technological development and Demonstration RTM Radiative Transfer Model SAGE Stratospheric Aerosol and Gas Experiment SAOZ Système d’analyse par observations zénithales SESAME Second European Stratospheric Arctic and Mid-latitude Experiment SCD Slant Column Density SCIAMACHY Scanning Imaging Absorption spectrometer for Atmospheric Chartography SYMOCS System for Monitoring Compounds in the Stratosphere SZA Solar Zenith Angle THESEO Third European Stratospheric Experiment on Ozone UKMO United Kingdom Meteorological Office UNEP United Nations Environment Programme VINTERSOL Validation of INTER-national Satellites and study of Ozone Loss VCD Vertical Column Density WMO World Meteorological Organisation WP Work package WWW World Wide Web (Internet) 1 1 QUILT Final Report I.3QUILT Final Report I.3 SECTION 6 Detailed report related to overall project duration QUILT - Final Report 47 Section 6: Detailed report related to overall project duration 6.1 Background In the Antarctic, springtime O3 started to decrease dramatically in the early 1980’s. From 1980 onwards the size of the “ozone hole”, defined as that region having O3 value below 220 DU, grew, and in addition it persisted for increasingly longer periods. After about 1989 the extent of depletion has stayed fairly constant with arguably some increase in size and duration. As a result of the halogen in- duced depletion cycles, it appears that throughout the 1990s, the stratospheric po- lar vortex air below approximately 25 km had very low values of ozone: the halogens being activated by the multi-phase or heterogeneous reactions of the so- called halogen reservoir species (eg. HCl, ClONO2, HOCl, BrONO2 etc.) on polar stratospheric clouds (PSCs), which form at relatively low stratospheric tempera- tures. The source of the halogens is primarily the anthropogenic release of chlo- rofluorocarbon compounds (CFCs), their substitutes, the HCFCs, the halons, methyl bromide and related compounds. The stratospheric dynamics above the Arctic in winter and spring is in comparison with that above Antarctica less pre- dictable. It also appears that the ozone depletion in the polar vortex seems to be somewhat time lagged compared to that above Antarctica. Several winters win- ters in the 1990s associated with a strong and persistent polar vortex in the North- ern Hemisphere and low temperatures have been characterised by severe Arctic ozone loss. Figure Figure 6.1.1-1 shows how the change from 1980 to 1997. Figure 6.1.1-1. Monthly mean total ozone over the Northern Hemisphere for the month of March for the years 1980 (left) and 1997 (right). Figures are based on data from TOMS on Nimbus-7 and on the Earth Probe. The in the 1970’s the typical behaviour of having an ozone “high” over the polar cap is in recent winters no longer the case. This pattern of stratospheric ozone be- haviour resembles that observed over Antarctica, although the depletion is less se- vere. In general the most significant cases of ozone loss have been associated with unusually low temperatures in the Arctic stratosphere. Examples of this behaviour were in the winters and springs of 1995/1996, 1996/1997 and it appears that 1999/ 2000 is following this pattern. In contrast the stratosphere had an early warming in 1997/1998 and 1998/1999, and consequently the polar vortex was not stable. Nevertheless halogen activation and some associated ozone depletion could be observed in the polar vortex. Recently a link between global warming and stratospheric ozone depletion has been made. The resultant cooling of the stratosphere appears to stabilise the polar vortex and increase ozone depletion. Simulations coupling general circulation Figure 6.1.1-2. GCM calculations coupled with stratospheric chemistry models with stratospheric chemistry predict that the total ozone in the Arctic vor- show than one can expect the ozone tex in March can drop to 120 DU during the decade from 2010 to 2019 [Shindell, minimum in March to drop down to et al. 1998]. This is illustrated in Figure 6.1.1-2. Although these calculations are around 120 DU in certain winters uncertain, they show that the coupling between ozone and climate can cause se- between 2010 and 2019. In Antarctica vere ozone depletion after the maximum in halogen loading has been reached. one could expect ozone to drop down to 75 DU. Adapted from Shindell et al. 48 QUILT - Final Report 6.1 Background At mid latitudes significant ozone depletion is also observed. The explanation of this phenomenon is not yet fully understood, having aspects of dilution of ozone poor air from the polar vortex, possible halogen activation and homogene- ous gas phase catalytic destruction of ozone by halogen species in the middle and upper stratosphere. Several other factors that remain yet to be explained include: ✎ The increasing trend of stratospheric NO2. The trend is larger than expected from decreasing aerosol loadings, and models also appear to under estimate NO2 in Polar Regions. ✎ What is the role of stratospheric iodine, i.e., IO, in stratospheric ozone depletion? In summary as a result of the non-linear complex nature of the atmosphere chemistry, transport models of the atmosphere in spite of large improvements in their accuracy in the last 25 years, continue to rely on the long-term measurement of atmospheric composition. For policymakers there is a clear need to continue and improve the accuracy of measurements of stratospheric constituents and com- bine these with models describing and predicting the behaviour of the strato- sphere. The growing risk for future ozone loss increases the need for reliable atmospheric chemistry models. The objectives of QUILT specifically address this need. QUILT aims to combine not only validated global measurements of ozone and key stratospheric constituents and test the capability of the atmospheric mod- els using existing data, but to extend and improve the data set for the period 2001 to 2003 when stratospheric halogen loading is predicted to reach its maximum. In addition it will continue to provide the user community with near real time data products from the European satellite instrument GOME. References: Shindell, D.T, D. Rind and P. Lonergan, Increased polar stratospheric ozone losses and delayed eventual recovery owing to increasing greenhouse-gas concentrations, Nature, 392, 589-592, 1998. WMO (1998), Scientific Assessment of Ozone
Details
-
File Typepdf
-
Upload Time-
-
Content LanguagesEnglish
-
Upload UserAnonymous/Not logged-in
-
File Pages181 Page
-
File Size-