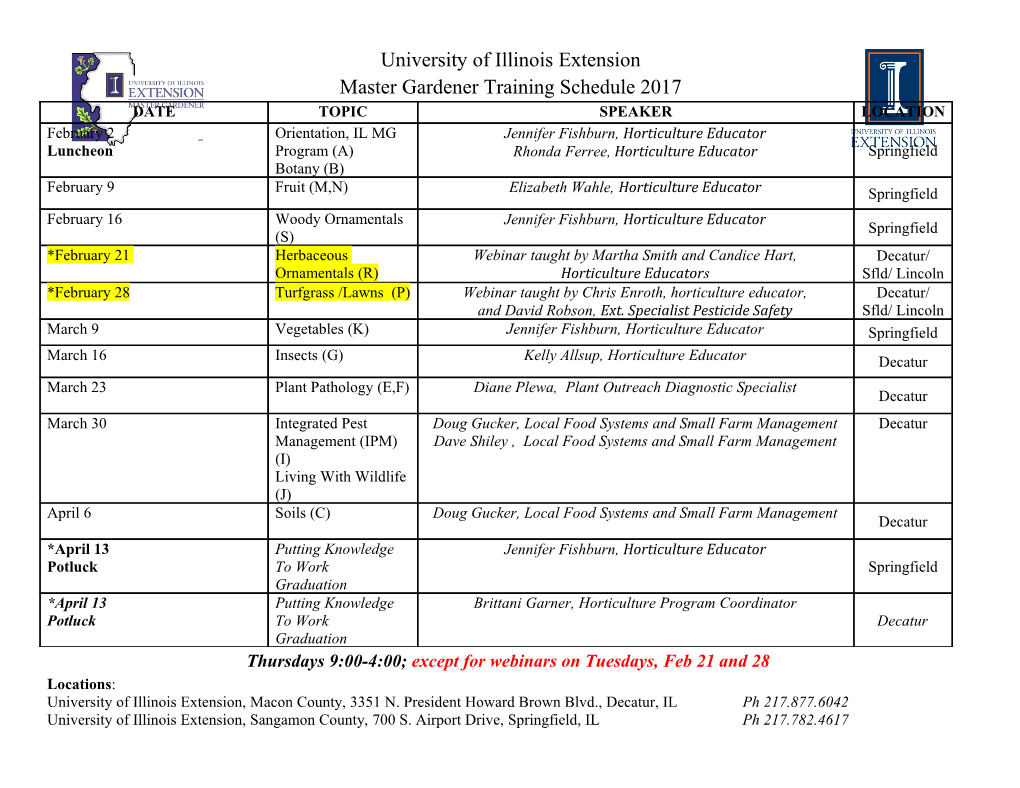
RESEARCH ARTICLE Analysis of Fault Zone Resonance Modes Recorded 10.1029/2020JB019756 by a Dense Seismic Array Across the San Jacinto Key Points: • Source‐independent resonance fault Fault Zone at Blackburn Saddle fi zone wave eld is consistently Hongrui Qiu1 , Amir A. Allam2 , Fan‐Chi Lin2,3 , and Yehuda Ben‐Zion4,5 observed after S wave arrival in data recorded by a dense array 1Department of Earth, Environmental and Planetary Sciences, Rice University, Houston, TX, USA, 2Department of • A fault zone model with a low 3 velocity layer between two quarter Geology and Geophysics, The University of Utah, Salt Lake City, UT, USA, Institute of Earth Sciences, Academia Sinica, spaces can fit well the resonance Taipei, Taiwan, 4Department of Earth Sciences, University of Southern California, Los Angeles, CA, USA, 5Southern wavefield at ~1.3 Hz California Earthquake Center, University of Southern California, Los Angeles, CA, USA • Modeling the resonance wavefield provides independent constraints on fault zone properties We present observations and modeling of spatial eigen‐functions of resonating waves within complementary to previous studies Abstract fault zone waveguide, using data recorded on a dense seismic array across the San Jacinto Fault Zone Supporting Information: (SJFZ) in southern California. The array consists of 5‐Hz geophones that cross the SJFZ with ~10–30 m • Supporting Information S1 spacing at the Blackburn Saddle near the Hemet Stepover. Wavefield snapshots after the S wave arrival are consistent for more than 50 near‐fault events, suggesting that this pattern is controlled by the fault zone structure rather than source properties. Data from example event with high signal to noise ratio show three Correspondence to: main frequency peaks at ~1.3, ~2.0, and ~2.8 Hz in the amplitude spectra of resonance waves averaged H. Qiu, [email protected]; over stations near the fault. The data are modeled with analytical expressions for eigen‐functions of [email protected] resonance waves in a low‐velocity layer (fault zone) between two quarter‐spaces. Using a grid search‐based method, we investigate the possible width of the waveguide, location within the array, and shear wave Citation: velocities of the media that fit well the resonance signal at ~1.3 Hz. The results indicate a ~300 m wide Qiu, H., Allam, A. A., Lin, F.‐C., & damaged fault zone layer with ~65% S wave velocity reduction compared to the host rock. The SW edge of ‐ Ben Zion, Y. (2020). Analysis of fault the low‐velocity zone is near the mapped fault surface trace, indicating that the damage zone is zone resonance modes recorded by a dense seismic array across the San asymmetrically located at the regionally faster NE crustal block. The imaging resolution of the fault zone Jacinto fault zone at Blackburn Saddle. structure can be improved by modeling fault zone resonance modes and trapped waves together. Journal of Geophysical Research: Solid Earth, 125, e2020JB019756. https://doi. org/10.1029/2020JB019756 Received 10 MAR 2020 1. Introduction Accepted 19 SEP 2020 Accepted article online 24 SEP 2020 Fault zones have hierarchical damage structures that include at places core low velocity layers that act as waveguides or trapping structures of seismic waves (e.g., Ben‐Zion & Sammis, 2003; Yang, 2015). Some elements of the core fault damage zone can have significant implications for ground motion predictions, properties of earthquake ruptures, and long‐term behavior of the fault. As examples, the velocity reduction in the fault zone can lead to considerable amplification of seismic waves (e.g., Kurzon et al., 2014; Rovelli et al., 2002; Spudich & Olsen, 2001), asymmetric damage zones with respect to the fault may be used to infer on preferred propagation direction of earthquake ruptures (e.g., Ben‐Zion & Shi, 2005; Dor et al., 2006), and low velocity damage zones can affect properties of earthquake sequences (e.g., Thakur et al., 2020). The clearest form of fault zone trapped waves (FZTW) is Love‐type signals associated with critically reflected phases that interfere constructively within the core damage zone (Ben‐Zion & Aki, 1990). These waves follow the S body wave with relatively high amplitude and low frequencies, are somewhat dispersive, and they exist predominantly in the vertical and fault parallel components of motion. Love‐type trapped waves have been recorded and analyzed at many fault and rupture zones in California (e.g., Catchings et al., 2016; Cochran et al., 2009; Lewis et al., 2005; Li et al., 1990, 1994; Peng et al., 2003; Yang et al., 2011), Japan (e.g., Mamada et al., 2004; Mizuno & Nishigami, 2006), Turkey (e.g., Ben‐Zion et al., 2003), Italy (e.g., Avallone et al., 2014; Rovelli et al., 2002), Israel (Haberland et al., 2003), and other locations. A less common type of trapped waves involves leaky modes (normal modes with phase velocities higher than body wave velocities) or Rayleigh‐type signals that appear on the radial and vertical components with appreciable amplitudes between the direct P and S waves (Gulley et al., 2017; Malin et al., 2006). These waves have been fi ©2020. American Geophysical Union. observed at the Park eld section of the San Andreas fault (e.g., Ellsworth & Malin, 2011) and several loca- All Rights Reserved. tions along the San Jacinto fault zone (e.g., Qin et al., 2018; Qiu et al., 2017). Data recorded recently by QIU ET AL. 1of21 Journal of Geophysical Research: Solid Earth 10.1029/2020JB019756 dense seismic arrays across fault zones enabled also construction of trapped waves from correlations of earthquake waveforms and ambient noise (Hillers & Campillo, 2018; Hillers et al., 2014; Wang et al., 2019). Normal modes are widely observed on Earth after large earthquakes (e.g., Block et al., 1970) or due to excita- tion by ocean waves (e.g., Webb, 2008). The energy generated by such sources excites the free oscillations of the earth and produces normal modes (i.e., standing interference pattern) that are only seen at specific eigen‐ frequencies and can be represented by a set of eigen‐functions (e.g., Gilbert, 1971). The observed eigen‐fre- quencies and eigen‐functions are sensitive to the earth interior structures and widely used to image the deep earth structures at a global scale (e.g., Dziewonski & Anderson, 1981). Normal modes can be found in any finite object, for example, freestanding rock arches (Geimer et al., 2020), when energy is trapped inside. Similarly, seismic energy that is trapped in a fault zone waveguide can also produce normal (or resonance) modes within the finite (both in width and depth) waveguide. The corresponding resonance eigen‐frequen- cies and eigen‐functions provide constraints on the internal structures of the fault zone waveguide. However, eigen‐frequencies and eigen‐functions of fault zone resonance waves have never been reported or analyzed so far, likely due to the limitation in seismic station coverage near major faults. The San Jacinto fault zone (SJFZ) is a major branch of the San Andreas fault system in southern California, and it accommodates a large portion of the plate boundary motion in the region (Fialko, 2006; Lindsey et al., 2014). The SJFZ has significant ongoing seismicity (Hauksson et al., 2012; Ross et al., 2017), and paleoseismic studies show that it is capable of producing large (Mw > 7.0) earthquakes (Rockwell et al., 2015, and references therein). To improve the knowledge on local earthquakes and the internal structure of the SJFZ, several seismic arrays were deployed in the last decade across different sections of the fault zone (e.g., Ben‐Zion et al., 2015; Li et al., 2019; Wang et al., 2019). Most arrays have relatively short aperture (~500 m) and station spacing of ~50 m. Since typical fault zone width ranges from 100 to 300 m for the SJFZ (Qin et al., 2018; Qiu et al., 2017; Share et al., 2017, 2019), it is hard to capture with such arrays the energy decay outside and free oscillations inside the fault zone waveguide. However, this may be done with data recorded by a ~2 km long array with instrument spacing of about 10–30 m at the Blackburn Saddle (BS) site of the SJFZ (Figure 1). In this study, we aim to investigate the existence and properties of fault zone resonance modes based on the data obtained by the dense array with relatively long aperture at the BS site. By closely examining waveforms for hundreds of regional earthquakes, we are able to robustly observe and confirm for the first time the presence of fault zone resonance modes. Analysis of the natural modal frequencies and eigen‐functions in the space‐time response of stations spanning the fault zone helps to constrain further properties of the fault zone waveguide, in addition to results based on waveform modeling of FZTW at individual stations. In the following we describe fundamental and first higher fault zone resonance modes observed at stations of the BS array that span the fault zone (section 3) and develop an analytical‐based methodology to infer key geometrical and seismic parameters from the observations (section 4). The modeling results are presented in section 5 and discussed in section 6. The observations and analyses augment the seismological techniques available for studying fault zone structures. 2. Data and Instrumentation We deployed a linear array of 108 Fairfield 3C 5‐Hz nodal seismometers recording continuously at 1,000 Hz sampling rate for 35 days (from 21 November 2015 to 26 December 2015) on the Clark segment of the SJFZ near the Hemet Stepover (Figure 1a; Allam, 2015). The deployment (BS01‐108) was approximately perpen- dicular (NE to SW) to the fault surface trace in Blackburn Saddle, with station BS55 closest to the mapped fault (Figure 1b). The array was deployed with station spacing that is ~10 m in a 400 m wide area centered on the mapped fault surface trace and ~30 m elsewhere.
Details
-
File Typepdf
-
Upload Time-
-
Content LanguagesEnglish
-
Upload UserAnonymous/Not logged-in
-
File Pages21 Page
-
File Size-