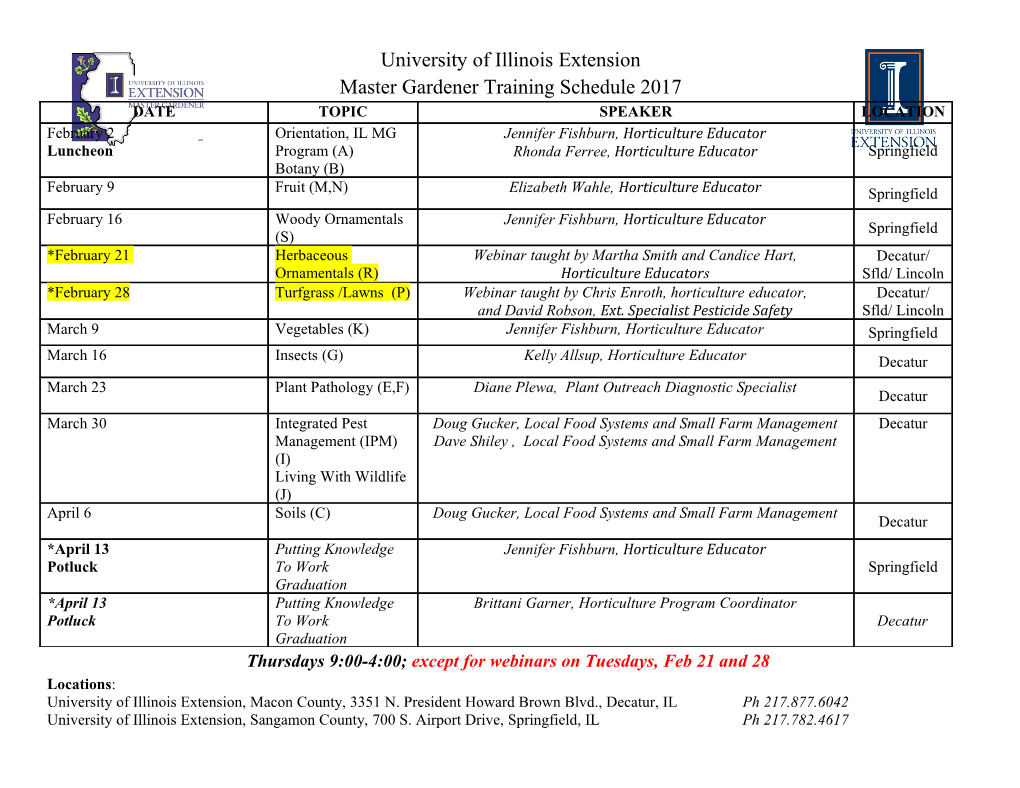
Wayne State University Wayne State University Theses 1-1-2016 Effects Of Folding On Mineral Cpos In Gneiss: Implications For Lower Crustal Seismic Anisotropy Alexia K. Sarnowsky Wayne State University, Follow this and additional works at: https://digitalcommons.wayne.edu/oa_theses Part of the Geology Commons Recommended Citation Sarnowsky, Alexia K., "Effects Of Folding On Mineral Cpos In Gneiss: Implications For Lower Crustal Seismic Anisotropy" (2016). Wayne State University Theses. 507. https://digitalcommons.wayne.edu/oa_theses/507 This Open Access Thesis is brought to you for free and open access by DigitalCommons@WayneState. It has been accepted for inclusion in Wayne State University Theses by an authorized administrator of DigitalCommons@WayneState. EFFECTS OF FOLDING ON MINERAL CPOS IN GNEISS: IMPLICATIONS FOR LOWER CRUSTAL SEISMIC ANISOTROPY by ALEXIA SARNOWSKY THESIS Submitted to the Graduate School of Wayne State University, Detroit, Michigan in partial fulfillment of the requirements for the degree of MASTER OF SCIENCE 2016 MAJOR: GEOLOGY Approved By: _______________________________ Advisor Date ACKNOWLEDGEMENTS I would like to thank my advisor Sarah Brownlee for allowing me to do research with her for a Masters in geology. I would like to thank Wayne State University for admitting me as a graduate student to obtain my Masters degree. I would also like to thank Bill Cambray for giving us the rock sample used in this study and my family and friends for helping me every step of the way in completing this thesis. ii TABLE OF CONTENTS Acknowledgements_____________________________________________________________ii Table of Contents______________________________________________________________iii List of Tables _________________________________________________________________v List of Figures________________________________________________________________vi Introduction___________________________________________________________________1 Geologic Background___________________________________________________________6 2.1 Appalachian Mountains ________________________________________________6 2.2 Coweeta Group_______________________________________________________6 Materials & Methods ___________________________________________________________8 3.1 Samples ____________________________________________________________8 3.2 Thin Section Preparation and Polishing____________________________________8 3.3 Petrography _________________________________________________________9 3.4 Electron Backscatter Diffraction (EBSD___________________________________9 3.5 Crystallographic Preferred Orientations___________________________________13 3.6 Elastic Tensor and Seismic Anisotropy Calculations_________________________13 Results______________________________________________________________________16 4.1 Geometry___________________________________________________________16 4.2 Composition ________________________________________________________17 4.3 Mineral CPOs _______________________________________________________18 4.3.1 CPO Strength________________________________________________18 4.3.2 Quartz______________________________________________________20 4.3.3 Feldspar ____________________________________________________21 iii 4.3.4 Biotite______________________________________________________30 4.3.5 Hornblende__________________________________________________30 4.4 Seismic Properties____________________________________________________30 4.4.1 Isotropic Properties ___________________________________________30 4.4.2 Anisotropy __________________________________________________31 4.4.3 Symmetry___________________________________________________34 Discussion___________________________________________________________________35 5.1 Hypothesis Testing ___________________________________________________35 5.2 Seismic Anisotropy___________________________________________________38 5.2.1 Variations Through The Fold____________________________________38 5.3 Implications for Modeling Structural Effects on Seismic Anisotropy ____________41 Conclusions__________________________________________________________________42 References___________________________________________________________________43 Abstract_____________________________________________________________________47 Autobiographical Statement_____________________________________________________48 iv LIST OF TABLES 4.1 _________________________________________________________________________18 4.2 _________________________________________________________________________20 4.3 _________________________________________________________________________31 4.4 _________________________________________________________________________34 5.1 _________________________________________________________________________40 5.2 _________________________________________________________________________40 v LIST OF FIGURES 1.1 __________________________________________________________________________5 2.1a _________________________________________________________________________7 2.1b _________________________________________________________________________7 3.1 _________________________________________________________________________11 3.2 _________________________________________________________________________12 4.1 _________________________________________________________________________17 4.2 _________________________________________________________________________22 4.3 _________________________________________________________________________24 4.4 _________________________________________________________________________26 4.5 _________________________________________________________________________28 4.6 _________________________________________________________________________32 vi 1 CHAPTER 1: INTRODUCTION Most of our knowledge of the interior structure of the Earth comes from interpretations of geophysical observations. These interpretations rely on accurate characterization of the geophysical properties of known materials. Density, bulk and shear moduli have been measured for a large number of rock types and single minerals at a variety of temperature and pressure conditions (Weiss, 1999; Mainprice & Nicolas, 1989). As a result, we understand quite well how a particular rock, or aggregate of known mineral proportions should appear at depth in terms of isotropic seismic velocities. When it comes to distinguishing mantle rocks from crust, lower crust from upper crust, or granite from basalt, isotropic seismic properties are quite effective. However, isotropic seismic properties are not sufficient to distinguish between many rock types believed to make up the middle and lower continental crust. Seismic anisotropy is the directional dependence of seismic velocity, and can have a number of causes including layering (Backus, 1962; Kern, 2008), aligned cracks (Kern et. al., 2008), and preferred crystallographic orientation of minerals (Weiss, 1999; Drury, 2011). If the causes of seismic anisotropy at the rock scale are known, we can use anisotropy to improve our understanding of the composition and structure of the middle and lower continental crust. Compared with the mantle, we know quite little about the composition and structure of the lower continental crust. The mantle has a relatively simple composition, containing much less mineralogic diversity than the crust, and a wealth of studies have focused on mineral structures and deformation at mantle pressure and temperature conditions (Blackman, 2002). As a result, we have a far better understanding of the seismic properties, including anisotropy, of the mantle than we do of the crust. 2 The main cause of anisotropy in the upper mantle is believed to be the preferred orientation of olivine, which develops as a result of activation of preferred mineral slip systems during deformation at particular conditions (Ji et al., 2004; Warren, 2008). The active deformation mechanisms in olivine have been well-studied using laboratory experiments (Ji et al., 2004; Warren, 2008), so interpreting flow directions from anisotropy as a result of olivine preferred orientation is relatively straightforward, and is becoming somewhat routine (Warren, 2008). The crust, on the other hand, is much more complex, but equally as important to understand. The crust makes up a tiny proportion of Earth’s mass (~0.6%), but due to its high concentration of refractory elements, the crust contains up to 70% of the total silicate Earth’s budget of important heat producing elements (Rudnick and Fountain, 1995). Models of the thermal structure of Earth thus rely on accurate characterization of the composition of the crust, including the lower crust. Previous studies that have attempted to use seismic properties to distinguish between lower crustal rock compositions have combined isotropic seismic properties with other geophysical and geochemical measurements with relatively limited success. High seismic velocities in the lower crust, in combination with lower than expected surface heat flow measurements for felsic compositions, have been interpreted as indicating a mafic lower crust (Rudnick and Fountain, 1995; Rudnick and Gao, 2003). However, there are felsic rocks that have the same high isotropic velocities, and interpretations of surface heat flow are highly dependent on the heat flux from the mantle, which is poorly constrained (Hacker et al., 2015). So even when combined with other observations, isotropic seismic properties cannot place significant constraints on the composition
Details
-
File Typepdf
-
Upload Time-
-
Content LanguagesEnglish
-
Upload UserAnonymous/Not logged-in
-
File Pages55 Page
-
File Size-