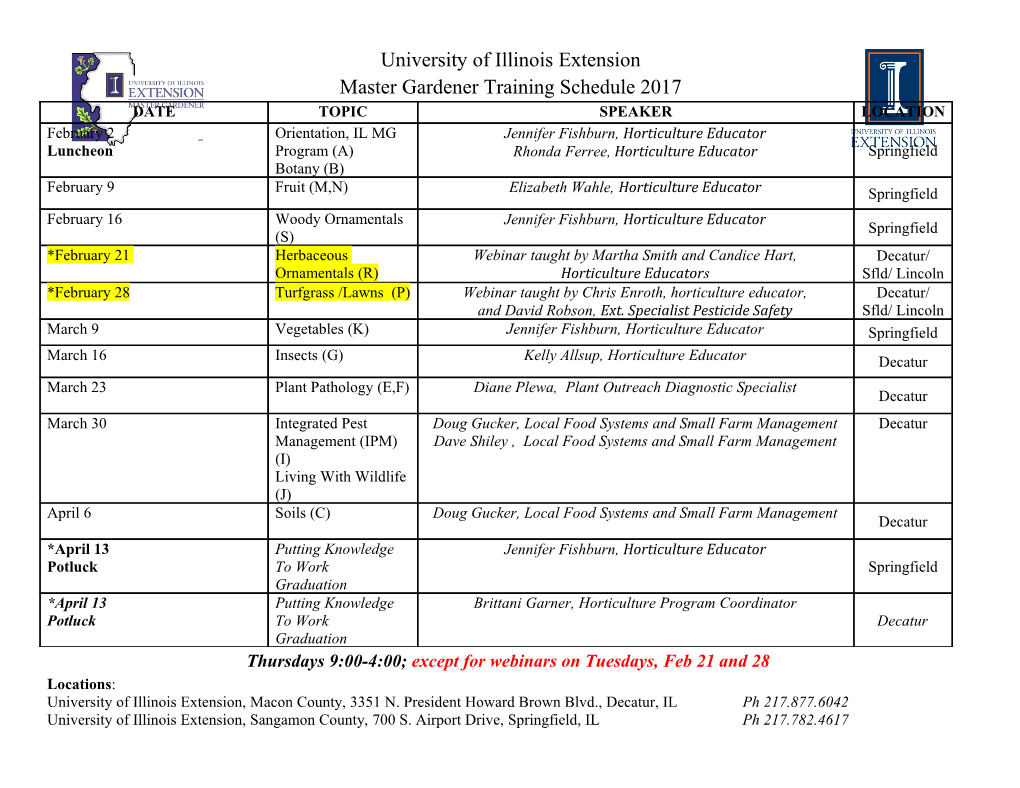
DEGREE PROJECT IN VEHICLE ENGINEERING, SECOND CYCLE, 30 CREDITS STOCKHOLM, SWEDEN 2017 A study of SPEs and the consequences of radiation exposure SIGNE BJÖRNHOLDT BÖLL KTH ROYAL INSTITUTE OF TECHNOLOGY SCHOOL OF ENGINEERING SCIENCES TRITA AVE 2017:15 ISSN 1651-7660 www.kth.se A study of SPEs and the consequenses of radiation exposure Author: Signe Björnholdt Böll [email protected] Royal Institute of Technology (KTH) Supervisors: Christer Fuglesang Oscar Larsson February 22, 2017 c Signe Björnholdt Böll, 2017 Till mamma och pappa. Abstract With thorough literature studies as well as simulations, a way to minimize the exposure to radiation that astronauts are at risk of encountering during a solar proton event is sought. The understanding of where these particles come from, as well as the random nature of solar particle events is of importance in order to predict their occurrence. Different models used for predicting solar particle events based on a Poisson possibility distribution are presented, as well as real-time forecasts which give a warning of an approaching event. Although the models used for real-time forecasts have a high accuracy rate, the average warning time is only approximately one hour. The downside with the predicted possible occurrence is that this only gives a statistical probability of events that could possibly occur. For the real-time forecasts the downside is that with an average warning time of only one hour, they do not give a lot of time for seeking shelter during the onset of an event. With simulations it is shown that the best way to minimize the radiation dose obtained by astronauts is to use different materials of shielding. It is also shown that a lower shielding thickness when encountering SPEs, for example when in a space suit, is useful as long as the total amount of time spent in this suit during the duration of a mission is planned thoroughly in order to stay below the radiation dose limits. If an astronaut would be caught in an event with the same magnitude and intensity as the solar particle event of August 1972, it is shown that the astronaut only has nine minutes to seek shelter before exceeding the radiation dose limits and thereby risking radiation induced sickness. Sammanfattning Med djupgående litteraturstudier, samt simuleringar, undersöks möjligheten att minimera den strålnings- dos som astronauter riskerar att utsättas för under en protonstorm från solen. Förståelse för varifrån dessa laddade partiklar kommer, och även deras slumpmässiga beteende, är av vikt för att kunna förutspå dessa fenomen. Diverse modeller som används för att förutsäga hur dessa solstormar sannolikt kommer inträffa i framtiden, tillsammans med modeller som presenterar en prognos i realtid, diskuteras. Även om de tillgängliga realtidsmodellerna har en hög noggrannhet, så är ändå varningstiden inför en solstorm i medel omkring endast en timme. Detta ger väldigt lite tid för att söka skydd om en protonstorm skulle inträffa. Nackdelen med de förutsägelser som bygger på sannolikhet är att det endast ger en indikation på vad för slags protonstormar som skulle kunna inträffa. Med simuleringar visas att det bästa sättet att minimera strålningsdosen är genom att använda olika material samt tjocklek för att skärma av och skydda astronauten mot inkommande strålning. Simuleringarna visar även att ett tunnare skydd, som tex av en rymddräkt, kan vara användbart så länge den totala tiden som spenderas i strålningsmiljö planeras varsamt och delas upp mellan olika skyddsmaterial. Detta för att ej överskrida gränsen för accepterad strålningsdos. Slutligen visas även att en astronaut som befinner sig i en protonstorm med samma intensitet som den protonstorm som inträffade i augusti 1972 endast har 9 minuter på sig att söka skydd innan den accepterade dosen av strålning är överskriden och risken för akut strålningssjuka ökar. Contents 1 Introduction 9 2 Understanding solar particle events 10 2.1 The theory of origin . 11 2.1.1 Type III bursts and impulsive SPEs . 13 2.1.2 Type II bursts and gradual SPEs . 14 3 Prediction of events 15 3.1 Long-term prediction . 15 3.1.1 The King model . 16 3.1.2 The JPL model . 17 3.1.3 The ESP model . 19 3.1.4 The Rosenqvist et al. model . 20 3.1.5 Comparing the models . 20 3.2 Short-term prediction . 23 3.2.1 The CREME models . 23 3.2.2 The ESP model . 24 3.2.3 Comparing the models . 25 3.3 Real-time forecasts . 29 3.3.1 UMASEP . 30 3.3.2 The Laurenza technique . 35 3.3.3 PROTONS . 35 3.3.4 The proton prediction system . 35 3.3.5 The Posner model . 36 3.3.6 The difference in forecasts . 36 3.4 Time of warning . 38 4 The impact of radiation on the human body 39 4.1 Gray vs Sievert . 40 4.2 The consequences of exposure . 43 5 Radiation dose for space missions 45 5.1 Mission 1: Living and working on the ISS . 46 5.2 Mission 2: Exploring the Moon . 48 5.3 Mission 3: Exploring Mars . 50 5.4 Obtained radiation during missions . 52 5.5 Radiation dose during the solar particle event in August 1972 . 55 6 Conclusion 61 1. Introduction Since 1843 it has been known that the Sun, being a dynamic source of energy with constant dramatic events taking place on its surface, follows a periodic cycle. The cycle follows the variation in number of sun spots on the Earth’s surface, where the occurrence of big solar flares and coronal mass ejections intensifies as the solar cycle maximum approaches [Schwabe, 1844]. As seen in table 1, the solar cycle is on average 11 years long and stretches from minimum to minimum. Table 1: List of solar cycles tracked since 1755, with a mean value of 11 years [Kane, 2002]. Cycle Started Finished Duration max. monthly (years) sunspot number Solar cycle 1 1755 August 1766 March 11.3 86.5 (June 1761) Solar cycle 2 1766 March 1775 August 9.0 115.8 (Sep 1769 Solar cycle 3 1775 August 1784 June 9.3 158.5 (May 1778) Solar cycle 4 1784 June 1798 June 13.7 141.2 (Feb 1788) Solar cycle 5 1798 June 1810 September 12.6 49.2 (Feb 1805) Solar cycle 6 1810 September 1823 December 12.4 48.7 (May 1816) Solar cycle 7 1823 December 1833 October 10.5 71.5 (Nov 1829) Solar cycle 8 1833 October 1843 September 9.8 146.9 (Mar 1837) Solar cycle 9 1843 September 1855 March 12.4 131.9 (Feb 1848) Solar cycle 10 1855 March 1867 February 11.3 98.0 (Feb 1860) Solar cycle 11 1867 February 1878 September 11.8 140.3 (Aug 1870) Solar cycle 12 1878 September 1890 June 11.3 74.6 (Dec 1883) Solar cycle 13 1890 June 1902 September 11.9 87.9 (Jan 1894) Solar cycle 14 1902 September 1913 December 11.5 64.2 (Feb 1906) Solar cycle 15 1913 December 1923 May 10.0 105.4 (Aug 1917) Solar cycle 16 1923 May 1933 September 10.1 78.1 (Apr 1928) Solar cycle 17 1933 September 1944 January 10.4 119.2 (Apr 1937) Solar cycle 18 1944 January 1954 February 10.2 151.8 (May 1947) Solar cycle 19 1954 February 1964 October 10.5 201.3 (Mar 1958) Solar cycle 20 1964 October 1976 May 11.7 110.6 (Nov 1968) Solar cycle 21 1976 May 1986 March 10.3 164.5 (Dec 1979) Solar cycle 22 1986 March 1996 June 9.7 158.5 (Jul 1989) Solar cycle 23 1996 June 2008 January 11.7 120.8 (Mar 2000) Solar cycle 24 2008 January Still ongoing - 81.9 (Apr 2014) Mean 11.1 114.1 In combination with these solar flares and coronal mass ejections, an acceleration of particles can sometimes occur with particles being thrown out into space from the Sun’s surface. Figure 1 shows daily sunspot number compared to solar activity and the space environment between 1983-2012, where it can be seen that the protons measured by GOES satellites follow the periodical cycle of the average number of sunspots, known as the solar cycle. 9 Figure 1: An overview of the space environment between 1983-2012 showing the daily Sunspot number compared to solar activity. From top to bottom the graphs illustrates the daily sunspot number, cosmic rays, measured X-rays, measured protons and the GOES magnetometer. [Wilkinson, 2012] These solar particle events, or SPEs, can be hazardous to both astronauts and spacecrafts in space. As travelling to Mars has become a soon possible reality, astronauts will spend more time in space making the risk of exposure to SPEs much higher. Therefore it is important to know more about SPEs, how and when they occur and also how to protect astronauts if they are exposed to them. The total radiation an astronaut is exposed to in space consists of both radiation from SPEs and protons, as well as the long-term radiation from the galactic cosmic ray. In low earth orbit there is also a radiation contribution from trapped particles in Earth’s magnetic field. This thesis will only focus on the radiation originating from SPEs built up by protons, where the aim of this thesis is to investigate the possibility to predict solar particle events in order to minimize the risk of exposure and to maximize the time of warning. Another aim of this thesis is to investigate the use of shielding as a way to minimize radiation on the human body. Literature studies of historical events, the possibility to predict future events, radiation impact on the human body as well as simulations of the radiation level for different materials of shielding will be performed in order to do so.
Details
-
File Typepdf
-
Upload Time-
-
Content LanguagesEnglish
-
Upload UserAnonymous/Not logged-in
-
File Pages67 Page
-
File Size-