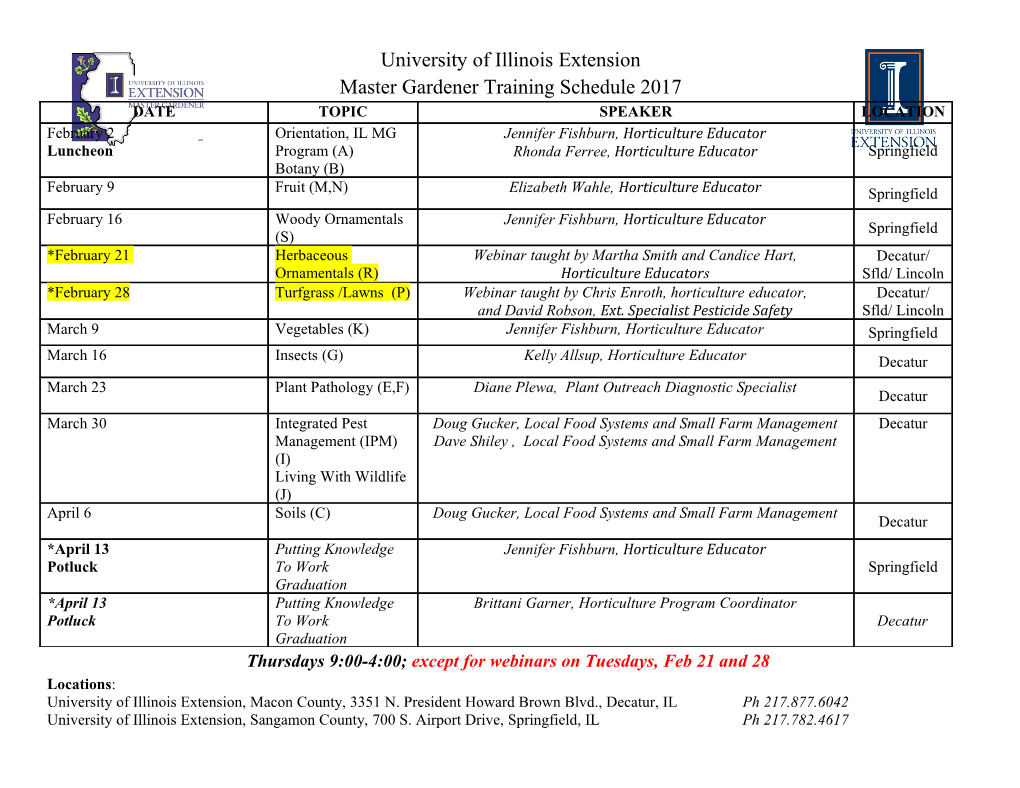
WIRELESS POWER TRANSFER: EFFICIENCY, FAR FIELD, DIRECTIVITY, AND PHASED ARRAY ANTENNAS by Abigail Jubilee Kragt Finnell A Thesis Submitted to the Faculty of Purdue University In Partial Fulfillment of the Requirements for the degree of Master of Science Department of Electrical and Computer Engineering Indianapolis, Indiana August 2021 THE PURDUE UNIVERSITY GRADUATE SCHOOL STATEMENT OF COMMITTEE APPROVAL Dr. Peter Schubert, Chair Department of Electrical and Computer Engineering Dr. Maher Rizkalla Department of Electrical and Computer Engineering Dr. Lauren Christopher Department of Electrical and Computer Engineering Approved by: Dr. Brian King 2 TABLE OF CONTENTS LIST OF TABLES ..................................... 5 LIST OF FIGURES .................................... 6 ABSTRACT ........................................ 7 1 INTRODUCTION ................................... 8 2 FUNDAMENTALS ................................... 10 2.1 Propagation of Signals and Power ........................ 10 2.1.1 The Friis Equation ............................ 11 2.1.2 The Goubau Equation .......................... 11 2.1.3 The Far Field Equation ......................... 12 2.2 Wireless Power Beaming ............................. 14 2.3 Gain and Directivity ............................... 15 2.3.1 Example: Transmit Antenna Trade-off Study ............. 17 2.4 Sidelobe Levels .................................. 18 2.5 Non-Traditional Phased Array Antenna Architecture ............. 21 2.6 Other Components of Wireless Power Transfer ................. 22 3 FAR FIELD DISTANCE STUDY ........................... 25 3.1 Far Field Model .................................. 26 3.2 MATLAB Model ................................. 27 3.3 Laboratory Work at IUPUI ........................... 33 4 FREE SPACE TRANSMISSION EFFICIENCY STUDY .............. 40 4.1 Efficiency Equations ............................... 41 Assuming a Constant D(φ) ....................... 42 Assuming D(φ) is Parabolic on a Logarithmic Scale .......... 43 Using Numeric Integration ........................ 44 4.2 Analysis of a Uniform PAA ........................... 45 4.3 Past WPT Experiments ............................. 47 4.3.1 The Microwave Powered Helicopter ................... 47 4.3.2 The JPL Experiment ........................... 49 3 4.3.3 The Goldstone Experiment ....................... 50 4.3.4 Equation Comparison .......................... 51 5 DISCUSSIONS AND ANALYSIS ........................... 53 6 CONCLUSIONS AND FUTURE WORK ....................... 55 6.1 Future Work ................................... 57 REFERENCES ....................................... 59 A MATLAB Code ..................................... 62 B Laboratory Data .................................... 73 4 LIST OF TABLES B.1 Lab Data: 1.45 m Distance ............................. 73 B.2 Lab Data: 1.2 m Distance .............................. 77 B.3 Lab Data: 0.84 m Distance ............................. 81 5 LIST OF FIGURES 2.1 Transmit Antenna Costs [14 ] ........................... 18 2.2 Grating Lobes for 1.5 λ Spacing, Zero Element Phase Shift .......... 20 2.3 Linear Phased Array Antenna, θ = p/4 ..................... 23 3.1 Far Field Square Rectenna Size vs. Distance .................. 28 3.2 Comparison of Singleton and 2x2 Array Phase at 125 mm .......... 29 3.3 Comparison of Singleton and 2x2 Array Phase at 154 mm .......... 29 3.4 Comparison of Singleton and 2x2 Array Phase at 600 mm .......... 30 3.5 Comparison of Singleton and 2x2 Array Phase at 1000 mm .......... 30 3.6 4x4 Antenna Array: 125 mm ........................... 31 3.7 4x4 Antenna Array: 160 mm ........................... 31 3.8 4x4 Antenna Array: 200 mm ........................... 31 3.9 4x4 Antenna Array: 350 mm ........................... 31 3.10 4x4 Antenna Array: 850 mm ........................... 32 3.11 4x4 Antenna Array: 2 m ............................. 32 3.12 Far Field Circular Rectenna Size vs. Distance ................. 33 3.13 Comparison of Traditional and Modeled Far Field for PAAs ......... 34 3.14 Laboratory Setup at IUPUI ........................... 35 3.15 Laboratory Setup ................................. 36 3.16 Comparison of Laboratory Results with MATLAB Data ........... 37 3.17 Far Field Model Comparison ........................... 39 4.1 Power Beaming Block Diagram ......................... 40 4.2 Layout Used for Efficiency Calculations ..................... 41 4.3 Maximum Directivity vs. Lambda Spacing ................... 46 4.4 Maximum Directivity vs. Number of Antennas ................ 47 4.5 Microwave Powered Helicopter Experiment [31 ] ................ 48 4.6 The JPL Experiment [33 ] ............................ 50 4.7 Goldstone Experiment [35 ] ............................ 51 4.8 Free Space Efficiency Equation Comparison .................. 52 6 ABSTRACT This thesis is an examination of one of the main technologies to be developed on the path to Space Solar Power (SSP): Wireless Power Transfer (WPT), specifically power beam- ing. While SSP has been the main motivation for this body of work, other applications of power beaming include ground-to-ground energy transfer, ground to low-flying satellite wireless power transfer, mother-daughter satellite configurations, and even ground-to-car or ground-to-flying-car power transfer. More broadly, Wireless Power Transfer falls under the category of radio and microwave signals; with that in mind, some of the topics contained within can even be applied to 5G or other RF applications. The main components of WPT are signal transmission, propagation, and reception. This thesis focuses on the transmission and propagation of wireless power signals, including beamforming with Phased Array An- tennas (PAAs) and evaluations of transmission and propagation efficiency. Signals used to transmit power long distances must be extremely directive in order to deliver the power at an acceptable efficiency and to prevent excess power from interfering with other RF technology. Phased array antennas offer one method of increasing the directivity of a transmitted beam through off-axis cancellation from the multi-antenna source. Besides beamforming, another focus of this work is on the equations used to describe the efficiency and far field distance of transmitting antennas. Most previously used equations, including the Friis equation and the Goubau equation, are formed by examining singleton antennas, and do not account for the unique properties of antenna arrays. Updated equations and evaluation methods are presented both for the far field and the efficiency of phased array antennas. Experimental results corroborate the far field model and efficiency equation presented, and the implications of these results regarding space solar power and other applications are discussed. The results of this thesis are important to the applications of WPT previously mentioned, and can also be used as a starting point for further WPT and SSP research, especially when looking at the foundations of PAA technology. 7 1. INTRODUCTION The number of papers regarding power beaming has increased significantly even as recently as in the year 2020. The reasons for this are numerous. Power beaming is seen as a field with increased potential at a time when transmitting and receiving antenna technologies are beginning to mature and WPT demonstrations are becoming more common. Additionally, international interest in SSP has increased; Japan, China, and the UK have all invested in SSP research. While the first inquiries into SSP were conducted in the 1970s, revived interest into this technology is unsurprising as alternate energy sources continue to be in high demand, and SSP has the unique capability of constant power, night and day, almost year-round. That being said, there are still many areas of SSP to be explored; much work on this subject is necessary before space solar power will be ready for wide-scale use. As mentioned above, much of the exploration into SSP (and consequently WPT) started in the early 1970s with interest from NASA. At the time, worldwide tensions about oil and energy garnered increased attention to renewable energy resources, and the idea of space solar power—while not original at the time—was considered as a potentially promising tech- nology. Some of the first experiments into power beaming were conducted in 1975 and developed with the help of William C. Brown at Raytheon, NASA, and JPL. In one ex- periment at Raytheon, an end-to-end efficiency of 54.18% was achieved at a distance of1.7 m. In another experiment at the JPL Goldstone facility later that year, Brown transferred 270 W over 1.54 km with a record-setting rectenna efficiency of over 80%. While this was a huge achievement, the large distance paired with the relatively small transmitting and receiving antenna resulted in a path loss of approximately 89%. As these experiments show, the main components and largest challenges of wireless power transfer have all been present from the beginning: incredibly high directivities are required to increase transmission effi- ciencies over long distances; safety, control, and careful evaluation are needed in all steps of the process; careful rectenna configurations are required for maximum energy harvesting; and beam steering is necessary for careful transmission. In addition to this, other compo- nents of WPT become relevant at high power densities: low sidelobe levels are required to 8 prevent the power level accessible to bystanders and external equipment from causing harm or interference,
Details
-
File Typepdf
-
Upload Time-
-
Content LanguagesEnglish
-
Upload UserAnonymous/Not logged-in
-
File Pages84 Page
-
File Size-