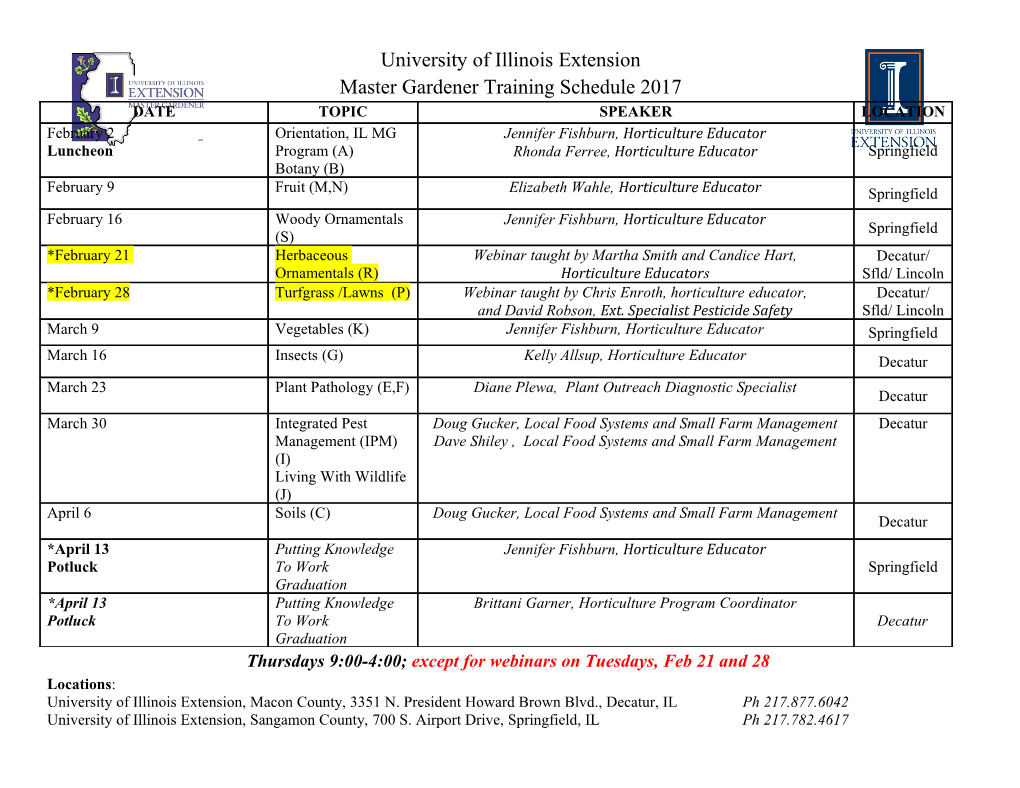
biomolecules Review Multifaceted Functions of Protein Kinase D in Pathological Processes and Human Diseases Xuejing Zhang , Jaclyn Connelly, Yapeng Chao and Qiming Jane Wang * Department of Pharmacology and Chemical Biology, University of Pittsburgh, Pittsburgh, PA 15261, USA; [email protected] (X.Z.); [email protected] (J.C.); [email protected] (Y.C.) * Correspondence: [email protected]; Tel.: +1-412-383-7754 Abstract: Protein kinase D (PKD) is a family of serine/threonine protein kinases operating in the signaling network of the second messenger diacylglycerol. The three family members, PKD1, PKD2, and PKD3, are activated by a variety of extracellular stimuli and transduce cell signals affecting many aspects of basic cell functions including secretion, migration, proliferation, survival, angiogenesis, and immune response. Dysregulation of PKD in expression and activity has been detected in many human diseases. Further loss- or gain-of-function studies at cellular levels and in animal models provide strong support for crucial roles of PKD in many pathological conditions, including cancer, metabolic disorders, cardiac diseases, central nervous system disorders, inflammatory diseases, and immune dysregulation. Complexity in enzymatic regulation and function is evident as PKD isoforms may act differently in different biological systems and disease models, and understanding the molecular mechanisms underlying these differences and their biological significance in vivo is essential for the development of safer and more effective PKD-targeted therapies. In this review, to provide a global understanding of PKD function, we present an overview of the PKD family in several major human diseases with more focus on cancer-associated biological processes. Citation: Zhang, X.; Connelly, J.; Chao, Y.; Wang, Q.J. Multifaceted Keywords: protein kinase D; diseases; cancer; cardiovascular diseases; CNS disorders; metabolic Functions of Protein Kinase D in diseases; inflammation; immune dysregulation Pathological Processes and Human Diseases. Biomolecules 2021, 11, 483. https://doi.org/10.3390/ biom11030483 1. Introduction Protein kinase D (PKD) was discovered near the turn of the second millennium, with Academic Editor: Peter Coopman PKD1 first reported in 1994 [1,2], followed by PKD3 in 1999 [3] and PKD2 in 2001 [4]. Due to the presence of a diacylglycerol (DAG)-binding C1 domain in its structure, PKD was Received: 2 February 2021 initially classified as an atypical protein kinase C (PKC) and given the name PKCµ for Accepted: 15 March 2021 ν Published: 23 March 2021 human PKD1 and PKC for PKD3. Later, recognizing the similarity of its catalytic domain to Ca2+/calmodulin-dependent protein kinases (CAMKs), PKD was reclassified into the Publisher’s Note: MDPI stays neutral CAMK group in the human kinome. Interestingly, PKD not only binds DAG but also with regard to jurisdictional claims in is activated by PKC through direct phosphorylation, a unique feature allowing PKD to published maps and institutional affil- integrate signal inputs from both DAG and PKC. iations. In the past two decades, extensive progress has been made towards the understanding of PKD structure, regulation, function, and signaling mechanisms. PKD has now emerged as a key signaling node in the DAG network, activated by a variety of cellular stimuli including growth factors, G protein-coupled receptor (GPCR) agonists, hormones, bioac- tive peptides, cellular stresses, and cytokines/chemokines, and coordinately regulates Copyright: © 2021 by the authors. Licensee MDPI, Basel, Switzerland. various downstream cellular processes, such as proliferation, survival, motility, secretion, This article is an open access article and gene expression. PKD resides or can be mobilized to different subcellular locations, distributed under the terms and including the plasma membrane, Golgi, mitochondria, and nucleus, to carry out unique conditions of the Creative Commons functions. Coinciding with its important roles in normal biology, dysregulation of PKD has Attribution (CC BY) license (https:// detrimental impacts and associates with a variety of pathological conditions and diseases creativecommons.org/licenses/by/ such as cancer, cardiac diseases, metabolic disorders, inflammatory diseases, neuronal 4.0/). dysfunctions, and immune dysregulation. Biomolecules 2021, 11, 483. https://doi.org/10.3390/biom11030483 https://www.mdpi.com/journal/biomolecules Biomolecules 2021, 11, 483 2 of 30 In this review, we outline past findings and new studies on PKD in various pathologi- cal conditions and diseases. Due to the space limitation, we only highlight the key findings that link PKD to specific diseases and their implications in therapy. In areas that have been more extensively studied, such as cancer, the discussion focuses on the diverse and often interconnected roles of PKD underlying the pathogenic processes of the disease. 2. PKD Structure and Regulation 2.1. Structure, Isoforms, and Expression/Tissue Distribution The family of PKD is evolutionarily highly conserved with three isoforms (PKD1, PKD2, and PKD3) identified in mammals. The three PKDs are similar in sizes (912, 878, and 890 amino acids (aa) for human PKD1, -2, and -3, respectively). PKD1 and PKD2 are most homologous and may originate from a common ancestor [5]. PKDs are widely distributed in the body, although their relative expression varies with different organs, with PKD1 and PKD2 being more prevalent in the lung, brain, kidney, heart, smooth muscle, pancreas, and prostate, and with PKD3 being more ubiquitous [1–4,6]. The conserved structure of PKD contains a N-terminal regulatory region that mainly comprises a C1 domain and a pleckstrin homology (PH) domain, followed by a C-terminal catalytic domain. The C1 domain constitutes two cysteine-rich Zn-finger-like motifs, Cla and Clb, that bind DAG and phorbol esters, the pharmacological analogs of DAG, with high affinity and regulate PKD localization to the nucleus, Golgi, and plasma membrane [7,8]. The PH domain exerts an autoinhibitory function on the catalytic domain to keep the kinase inactive at basal state [9,10]. Additionally, the regulatory domain contains an alanine–proline rich (AP) region at the N-terminus for PKD1 and a proline-rich (P) region for PKD2, and an acidic amino-acid-rich region (AD) between Clb and PH domains, and the functions of these domains remain obscure [11]. Recently, a ubiquitin-like domain (ULD) shared by all three PKD isoforms was identified at the N-terminus following the AP or P region. Based on the X-ray crystal structure of the ULD-C1a domain in the C. elegans PKD homolog DKF-1, ULD may act to initiate PKD dimerization at the membrane for trans-autophosphorylation at the activation loop in response to increased DAG concentration, leading to PKD activation possibly independent of PKC [5,12]. This domain appears to be conserved in all three human PKD isoforms [5,12]. The structure of PKD1 and PKD2 also contains a C-terminal PDZ domain that is thought to facilitate protein substrate recognition [7]. Within the PDZ domain, there is an autophosphorylation site (S910 for PKD1, S876 for PKD2), which is commonly used as a measure for PKD activation status, although its phosphorylation likely Biomolecules 2021, 11, alsox FOR PEER plays REVIEW a functional role [7,13,14] (see Figure1 for a schematic diagram3 of 33 of human PKD1, -2, and -3). Figure 1. A diagramFigure illustrating 1. A diagram the illustrating conserved the conserved structural structural domains domain ands and major major phosphorylation sites in sites human in protein human protein kinase D kinase D (PKD) isoforms. The structure of PKD contains a newly identified ubiquitin-like domain (ULD) for dimerization, (PKD) isoforms. Thea C1 structure domain (Cla of and PKD Clb) contains that binds diacylglycerol, a newly identified a pleckstrin ubiquitin-like homology (PH) domain domain for autoinhibition, (ULD) for dimerization,a catalytic a C1 domain domain for substrate phosphorylation, and a PDZ domain in PKD1 and PKD2 for protein interactions. Other domains with less known functions are the acidic amino-acid-rich region (AR) and an alanine–proline-rich region (AP) for PKD1 and a proline-rich region (P) for PKD2. Major phosphorylation sites and the upstream kinases that confer the phosphory- lation are indicated as well as the nuclear export signal (NES) and nuclear localization signal (NLS) for PKD2. Abbrevia- tions: trans-Golgi network (TGN), Abelson murine leukemia viral oncogene homolog 1 (Abl), casein kinase 1 (CK1). 2.2. Mechanisms of Regulation PKD can be activated downstream of GPCRs or receptor tyrosine kinases (RTKs) by a variety of stimuli such as hormones, growth factors, neuropeptides, lipids, and cellular stresses [7]. In a canonical activation pathway, following receptor stimulation, phospho- lipase Cs (PLCs) are activated to hydrolyze phosphatidylinositol 4,5-biphosphate (PIP2) to generate inositol 1,4,5-trisphosphate (IP3) and DAG. IP3 mobilizes internal calcium and DAG along with calcium (for cPKC) binds and anchors classic or novel protein kinases C (c/nPKC) to the plasma membrane and triggers their activation. DAG also recruits cyto- solic PKD to the plasma membrane by binding to its C1 domain; this process may induce a conformational change that allows PKC to colocalize with PKD at the plasma membrane to transphosphorylate a conserved serine residue (Ser738 for PKD1, Ser706 for PKD2, Ser731 for PKD3) in the activation loop of PKD, leading to the autophosphorylation of an adjacent serine
Details
-
File Typepdf
-
Upload Time-
-
Content LanguagesEnglish
-
Upload UserAnonymous/Not logged-in
-
File Pages30 Page
-
File Size-