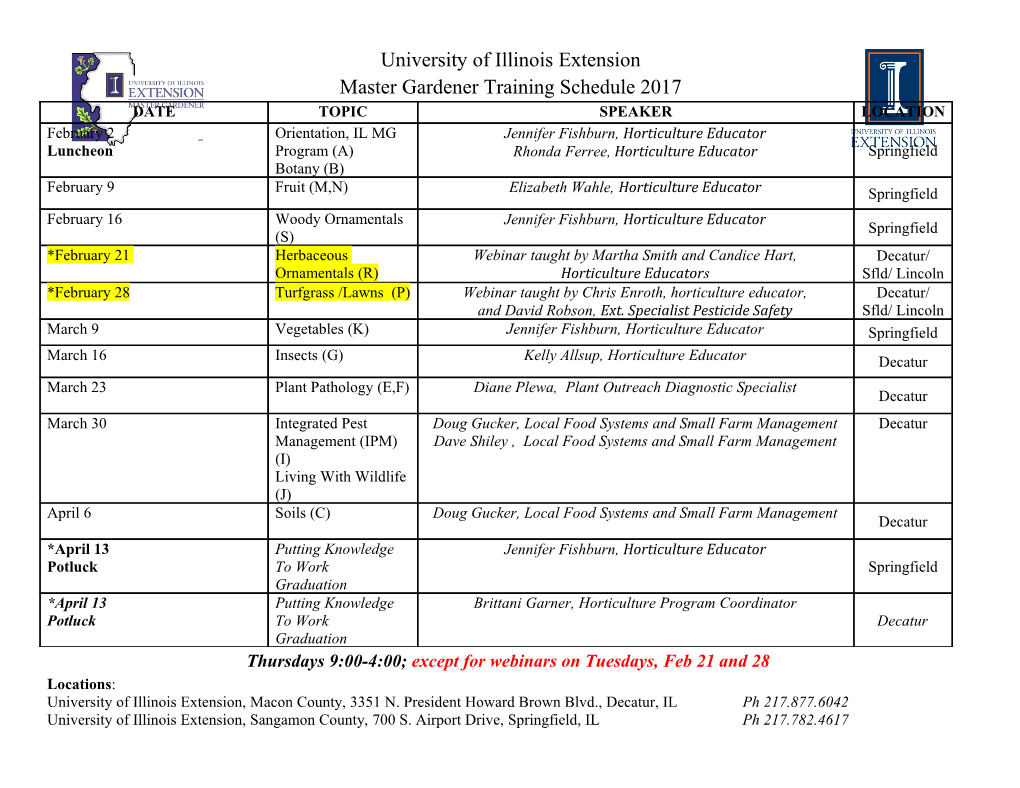
From protostars to adolescence: A tour of young stellar systems Gregory J. Herczeg (沈雷歌 Shěn Léigē ) Kavli Institute for Astronomy and Astrophysics, Peking University Photo by PhD student Ma Chao The last astrophysical step of our origins ? ? Serpens star-forming region Courtesy Adam Block via APOD Creating a picture of star-forming regions • Cold ISM: dust/gas (diffuse to dense) • Protostars • Adolescent stars Serpens star-forming region and Aquila Rift Courtesy Adam Block via APOD Far-IR/sub-mm: emission from dust and protostars Herschel far-IR dust image, Bontemps et al. 2010 Serpens Molecular Cloud 2 deg/15 pc Cambresy 2003 extinction map Serpens Molecular Cloud 2 deg/15 pc Add embedded protostars (yellow) and disks (red) from Spitzer, near-IR, X-ray Kuhn+2010; Povich+2013; Dunham+2015 Serpens Molecular Cloud 2 deg/15 pc Add optical counterparts from Gaia DR2 astrometry (Herczeg, Kuhn, et al. 2019) Protostars: ~few 105 yr Stellar growth Disks ~few 106 yr Planet formation Low mass stars, van Boekel 2005 Luminosity problem: how do stars grow? (JCMT Transient) Looking for planets inside disks Uncertainties in pre- main sequence stellar evolution Low mass stars, van Boekel 2005 Stars grow during Ltot=Lacc+Lphot protostellar phase Buried in envelope, Scattered by dust Cartoon from Isella 2006 Cartoon from Tobin+2012 Measure: Tbol (SED peak) and Lbol Measure Tbol (~peak of SED) and Lbol (luminosity) 1±480 ° 0.65 540 0.45 1 2±000 ° ° 0.25 060 Jy beam Dec (J2000) 0.05 120 -0.15 180 31m12s 30m24s 29m36s 18h28m48s RA (J2000) EnoCh+2009 Luminosity Problem (Kenyon et al. 1990; Dunham et al. 2010) Evolutionary Class No disk Disk Class I Class 0 Classical infall models: shaded region BolometriCLuminosity BolometriC Temperature Episodic bursts of accretion? (Kenyon et al. 1990; Dunham, Evans, et al. 2009) Steady accretion Episodic accretion Time dependenCe needed; episodiC aCCretion is likely (but not only) solution (e.g., Offner & McKee for different assumptions; FisCher+2017 for exponential deCay) Stars grow during bursts at young ages Tobin+2012 Zhu+2010; Bae+2014 FUor and EXor outbursts: discovered from optical monitoring (adapted from Kospal+2011) DeCades-long FUor bursts: Gravitational instabilities in disk Months-long EXor bursts MagnetiC instabilities in inner disk Most reCent bursts found by PTF/ZTF and Gaia The East Asian Observatory JCMT-Transient Survey: the first long-term sub-mm monitoring program (Herczeg+2017) Gregory Herczeg (co-PI; PKU/China) Yong-hee Lee (KHU), Wen-Ping Chen (NCU), Carlos Contreras-Pena (Exeter), Giseon Baek (KHU), Vivien Doug Johnstone (co-PI; NRC/Canada) Chen (NTHU), Jenny Hatchell (Exeter), Geoff Bower Jeong-Eun Lee (KHU/Korea) (EAO), Zhiwei Chen (PMO), Keping Qiu (NJU), Steve Mairs (EAO/Victoria) Jianjun Zhou (XAO) and ~70 others (Open team) The EAO/JCMT Transient Survey 7 SIPCA 2018 Expansion in 2020 to include2021-03 6- 17intermediate mass regions 18 JCMT-Transient Light Curves (Mairs+2017ab; Johnstone+2018; Lee et al. in prep) Precision of ~2% from Analysis of light curves “differential photometry” stochastic (random); secular (linear); and periodic SIPCA 2018 2021-03-17 19 Young Faithful: bursts and decays (Y-H Lee, Johnstone, et al. 2020; Contreras-Pena et al. 2020) Decay of accretion bursts (Y-H Lee, Johnstone, et al. 2020) Rise: 0.1 yr Decay: 0.75 yr Summary of variability over 4 years (Y-H Lee, Johnstone, et al. in prep) ) yr Fractional slope (/ slope Fractional Peak Flux (Jy/beam) Follow-up with NEOWISE mid-IR monitoring and Gemini spectroscopy Most luminous coronal radio flare ever detected? (Mairs+2019; Lalchand+ in prep for statistics) First coronal flare detected in sub-mm Decay detected during 30-min integration JW 566 (binary) in Orion: bright in a single epoch SIPCA 2018 2021-03-17 24 Future of JCMT-Transient Continued monitorinG of eiGht primary reGions Added 6 intermediate-mass star-forminG reGions Many more sources; confusion Only current method to detect protostellar variability on youngest protostars (Yoon, HerczeG, et al. in prep) Disk evolution and planet formation Low mass stars, van Boekel 2005 How would a forming planet affect a disk? Transitional(e.G., Lin Disks:& Papaloizu Gap1986; opening Zhu+2011) by planets? Zhu et al. 2011 Competition between Gas accretion; Gap/hole formation Tuesday, August 19, 14 Dust and gas disk timescales: planet formation must occur within ~3 Myr Disk frequency in different clusters (Hernandez et al. 2008 and many others, including Haisch+2001) Do disks show evidence of planet formation? Inner holes in dust Espaillat+2007; see also, eg Strom+1989, Brown et al. 2008 Calvet+2002, D’Alessio+2005, Furlan+2006; Kim+2009, Merin+2010 Atacama Large Millimeter Array (ALMA) Sub-mm interferometer, 5000m hiGh plateau in Chile Complete surveys of disk flux (~mass) e.g., Ansdell+2016; Pascucci+2016; Barenfeld+2016; see also Miotello+2017 and Long, Herczeg+2017 for CO flux From Long+2018a Complete surveys of disk flux (~mass) Cha I : 2 Myr-old star-forming region Long+2018a; see also, eg, Ansdell+ and Miotello+ Dust Mass Not enough to form gas giants ? Minimum mass Gas solar nebula Mass Not enough disk mass to grow more giant, terrestrial planets and to drive ratio accretion (see also Manara+; Mulders+) depends on dust opacities/scattering (Kataoka+2015; Zhu+2019) IRS 48: full gas ring revealed The ALMACycle 2 revolution:data Band 9 (van der Marel PI) Dust structures in protoplanetary disks 0.44 mm cont 13CO 6-5 Large dust grains drift to local pressure maxima (e.G., WeidenschillinG 1977; Pinilla+2015) Van der Marel et al. in prep - Dust trap optically thick, blocking some of 13CO emission? Fig. 1. Thermal- dust Kinematics emission fromof gas the around protoplanetary dust trap disk can surrounding test vortex Eliasmodel 2-27. The disk was imaged at a wavelength of 1.3 mm with ALMA reaching an angular resolution of 0.2600 0.2200 (indicated by the ellipse in the bottom-left corner), which corresponds to 36 ⇥ ⇥ 31 AU at the distance of the star (where AU is the astronomical unit). The field of view center (at 0,0) corresponds to the disk emission peak located at Right Ascension (J2000) = 16h 26m 45.024s, Declination (J2000) = –24d 23m 08.250s and coincidental with the position of the star Elias 2-27. (A) 1.3 mm dust continuum image from the Elias 2-27 protoplanetary disk over a 400 400 area. The color-scale represents flux density measured in units of Jansky per beam ⇥ 26 2 1 (1 Jy =10− Wm− Hz− ). (B) Increased contrast image from processing the original ALMA observations shown in panel (A) with an unsharp masking filter (17). 9 ALMA Image of HL Tau disk (0.5-1 Myr) (ALMA Partnership+2015) 2 scattered light emission (Akiyama et al. 2015; Rapson et al. 2015; Debes et al. 2013, 2016). In this Letter, we present and analyze observations that shed new light on the substructure in the TW Hya disk. We have used the long baselines of ALMA to mea- sure the 870 µm continuum emission from this disk at an unprecedented spatial resolution of 1 AU. Section 2 ⇠ presents these observations, Section 3 describes a broad- brush analysis of the continuum data, and Section 4 con- siders potential interpretations of the results in the con- texts of disk evolution and planet formation. 2. OBSERVATIONS AND DATA CALIBRATION TW Hya was observed by ALMA on 2015 Novem- ber 23, November 30, and December 1. The array in- cluded 36, 31, and 34 antennas, respectively, configured TW Hya (10 Myr) to span baseline lengths from 20 m to 14 km. The corre- Andrews+2016 lator processed four spectral windows centered at 344.5, Figure 1. Asynthesizedimageofthe870µmcontinuumemission 345.8, 355.1, and 357.1 GHz with bandwidths of 1875, from the TW Hya disk with a 30mas FWHM (1.6AU) circular beam. The RMS noise level is 35 µJy beam 1.Theinsetshows ⇠ − 469, 1875, and 1875 MHz, respectively. The observa- a0.200-wide (10.8 AU) zoom using an image with finer resolution (24 18 mas, or 1.3 1.0 AU, FWHM beam). tions cycled between the target and J1103-3251 with a ⇥ ⇥ 1 minute cadence. Additional visits to J1107-3043 were made every 15 minutes. J1037-2934, J1058+0133, and of 0.5–1 mm. The combined on-target integration time J1107-4449 were briefly observed as calibrators. The pre- was 95 minutes. The basic calibration was as described cipitable water vapor (PWV) levels were 1.0 mm on above. As a check, we compared the amplitudes from ⇠ November 23 and 0.7 mm on the latter two executions. each individual dataset on overlapping spatial frequen- The total on-target integration time was 2 hours. cies and found exceptional consistency. ⇠ These raw data were calibrated by NRAO sta↵. After The calibrated visibilities from each observation were applying phase corrections from water vapor radiometer shifted to account for the proper motion of the target measurements, the data were time-averaged into 2 s inte- and then combined after excising channels with potential grations and flagged for problematic antennas and times. emission from spectral lines. Some modest improvements The bandpass response of each spectral window was cal- were made with a round of phase-only self-calibration. ibrated using the observations of J1058+0133. The am- Continuum images at a mean frequency of 345.9 GHz plitude scale was determined from J1037-2934 and J1107- (867 µm) were generated by Fourier inverting the visi- 4449. The complex gain response of the system was cal- bilities, deconvolving with a multi-scale, multi-frequency ibrated using the frequent observations of J1103-3251. synthesis version of the CLEAN algorithm, and then restor- Although images generated from these data are relatively ing with a synthesized beam.
Details
-
File Typepdf
-
Upload Time-
-
Content LanguagesEnglish
-
Upload UserAnonymous/Not logged-in
-
File Pages62 Page
-
File Size-