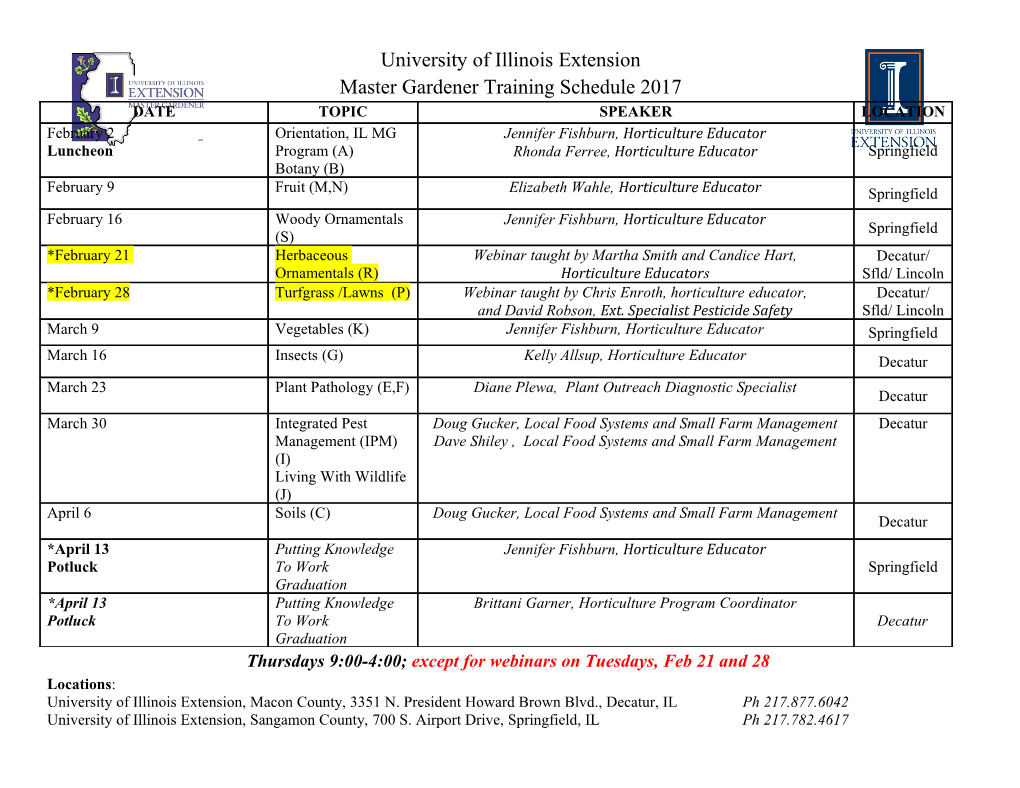
Solid Earth, 9, 879–896, 2018 https://doi.org/10.5194/se-9-879-2018 © Author(s) 2018. This work is distributed under the Creative Commons Attribution 4.0 License. Generating porosity during olivine carbonation via dissolution channels and expansion cracks Tiange Xing1, Wenlu Zhu1, Florian Fusseis2, and Harrison Lisabeth1,3 1Department of Geology, University of Maryland, College Park, MD, USA 2School of Geosciences, University of Edinburgh, Edinburgh, UK 3Department of Geophysics, Stanford University, Stanford, CA, USA Correspondence: Tiange Xing ([email protected]) Received: 2 April 2018 – Discussion started: 13 April 2018 Revised: 20 June 2018 – Accepted: 26 June 2018 – Published: 13 July 2018 Abstract. The olivine carbonation reaction, in which car- tion is self-sustained. Large fractures were generated as the bon dioxide is chemically incorporated to form carbonate, reaction proceeded and eventually disintegrated the aggre- is central to the emerging carbon sequestration method us- gate after 140 h. Detailed analysis show that these are expan- ing ultramafic rocks. The rate of this retrograde metamor- sion cracks caused by the volume mismatch in the cup walls, phic reaction is controlled, in part, by the available reactive between the expanding interior and the near-surface which surface area: as the solid volume increases during carbon- keeps a nearly constant volume. Nanotomography images of ation, the feasibility of this method ultimately depends on the reacted olivine cup reveal pervasive etch pits and worm- the maintenance of porosity and the creation of new reac- holes in the olivine grains. We interpret this perforation of tive surfaces. We conducted in situ dynamic X-ray micro- the solids to provide continuous fluid access, which is likely tomography and nanotomography experiments to image and key to the complete carbonation observed in nature. Reac- quantify the porosity generation during olivine carbonation. tions proceeding through the formation of nano- to micron- We designed a sample setup that included a thick-walled cup scale dissolution channels provide a viable microscale mech- (made of porous olivine aggregates with a mean grain size of anism in carbon sequestration practices. For the natural peri- either ∼ 5 or ∼ 80 µm) filled with loose olivine sands with dotite carbonation, a coupled mechanism of dissolution and grain sizes of 100–500 µm. The whole sample assembly was reaction-induced fracturing should account for the observed ◦ reacted with a NaHCO3 aqueous solution at 200 C, under self-sustainability of the reaction. a constant confining pressure of 13 MPa and a pore pressure of 10 MPa. Using synchrotron-based X-ray microtomogra- phy, the three-dimensional (3-D) pore structure evolution of the carbonating olivine cup was documented until the olivine 1 Introduction aggregates became disintegrated. The dynamic microtomog- raphy data show a volume reduction in olivine at the be- Mantle peridotites are exposed widely on the Earth’s sur- ginning of the reaction, indicating a vigorous dissolution face in tectonic settings such as mid-ocean ridges, subduction process consistent with the disequilibrium reaction kinetics. zones and ophiolites (Escartín et al., 1997; Fryer et al., 1995). In the olivine cup with a grain size of ∼ 80 µm (coarse- Peridotite is mainly composed of olivine which is unstable at ◦ grained cup), dissolution planes developed within 30 h, be- temperatures below 700 C in the presence of water (Evans, ◦ fore any precipitation was observed. In the experiment with 1977) and below 500 C in the presence of CO2-rich fluids the olivine cup of ∼ 5 µm mean grain size (fine-grained cup), (Johannes, 1969). The transformation of olivine to serpentine idiomorphic magnesite crystals were observed on the surface and carbonates due to fluid–rock interaction is extensively of the olivine sands. The magnesite shows a near-constant observed in peridotite outcrops (e.g., Beinlich et al., 2012; growth throughout the experiment, suggesting that the reac- Falk and Kelemen, 2015; Hansen et al., 2005). Rock defor- mation experiments have demonstrated that fluid alteration to Published by Copernicus Publications on behalf of the European Geosciences Union. 880 T. Xing et al.: Generating porosity during olivine carbonation peridotite can strongly affect the strength and tectonics of the peridotites cross-cutting hierarchical fracture networks filled oceanic lithosphere (Deschamps et al., 2013; Moore et al., by synkinematic carbonate and quartz veins extend to mi- 1996). Therefore, study of the olivine–fluid interaction is of croscopic scales. These cross-cutting networks indicate co- great importance for understanding the alteration process of eval carbonate crystallization and fracturing. Several studies peridotite in a variety of tectonic regions. General peridotite also showed that the forces generated by the volume increase alteration reactions can be formulated as follows (Hansen et should be enough to fracture peridotite (Iyer et al., 2008; al., 2005; Kelemen and Matter, 2008): Jamtveit et al., 2009, 2011; Ulven et al., 2014). While reaction-induced fracturing is accepted as a way Olivine C CO in fluid D Magnesite C Quartz; 2 to maintain fluid access, the mechanical details of the pro- Mg2SiO4 C 2CO2 D 2MgCO3 C SiO2; (R1) cess are poorly understood. As for the mechanism that gen- Olivine C CO2 in fluid C H2O D Talc C Magnesite; erates stresses, “crystallization pressure” (also termed “force of crystallization”) has been proposed (e.g., Scherer, 2004; 4Mg2SiO4 C 5CO2 C H2O D Mg3Si4O10.OH/2 Weyl, 1959; Winkler and Singer, 1972). In this model, C 5MgCO ; (R2) 3 the precipitation/crystallization of reaction products exert Olivine C H2O D Serpentine C Brucite; pressure around the growing crystals, and fracturing takes 2Mg2SiO4 C 3H2O D Mg3Si2O5.OH/4 C Mg.OH/2; (R3) place when that pressure exceeds the local minimum prin- cipal stress (Kelemen and Hirth, 2012). Salt crystallization Brucite C CO2 D Magnesite C H2O; (Scherer, 2004) is a common example where high crystal- Mg.OH/ C CO D MgCO C H O: (R4) 2 2 3 2 lization forces due to the nucleation of precipitates in pore Although peridotite weathering reactions occur widely in space cause samples to “burst from the inside” (see Fig. 1a). nature, the rate of olivine carbonation at subsurface condi- However, studies have shown that the crystallization force tions is debated. Since the retrograde metamorphic reactions is low in the olivine carbonation system (e.g., van Noort are kinetically fast, the extent of transformation is limited et al., 2017). Because of the lack of experimental evidence by fluid supply which depends on the accessible fluid path- of crystallization forces during olivine alteration, Zhu et ways. As the hydration and carbonation of olivine results in al. (2016) proposed an “expansion cracking” mechanism as an up to ∼ 44 % increase in solid molar volume (Goff and an alternative model after successfully producing reaction- Lackner, 1998; Hansen et al., 2005; Kelemen and Matter, induced fractures in an in situ synchrotron X-ray microto- 2008), carbonation of olivine is generally assumed to be self- mography study. In the expansion cracking model, tensile limiting: the reaction products would gradually fill up the stresses are generated due to the volume mismatch between pore space and lead to a decrease in the porosity (Emmanuel regions with different precipitation rates, leading to cracks and Berkowitz, 2006; Hövelmann et al., 2012), which in turn forming in regions that expand slower than their surround- lowers permeability and reduces fluid supply. This negative ings (see Fig. 1b). feedback would ultimately force the alteration to cease. How- Beyond fracturing, dissolution has been recognized as an ever, naturally occurring completely carbonated peridotites important part of the olivine alteration process (e.g., Vel- are evidence that these limitations can be overcome. For in- bel, 2009; Velbel and Ranck, 2008; Wilson and Jones, 1983) stance, listvenite is the natural completely carbonated prod- and proposed as a mechanism to explain the observed com- uct of peridotite, which is composed of magnesite, quartz plete carbonation of peridotite. In 1978, Grandstaff showed and trace minerals (Beinlich et al., 2012; Nasir et al., 2007). that dissolution could significantly increase the surface area This creates a conundrum of how the large extent carbonation through etch pitting. Wilson (2004) suggested that the weath- can be achieved with the potential self-limitation of reducing ering of olivine is controlled by etch pitting and channel for- fluid pathways. mation due to preferential dissolution, which assists the mi- In order to explain this discrepancy between the theory and gration of fluid and promotes further reaction. Andreani et the observation, numerous studies have been conducted aim- al. (2009) suggested that permeability may be maintained ing to find a mechanism to maintain the access for reacting during peridotite carbonation by the development of prefer- fluid during olivine alteration reactions. In 1985, Macdon- ential flow zones. Lisabeth et al. (2017a, b) observed relevant ald and Fyfe examined naturally altered peridotite and pro- structures in dunite samples that have been reacted under posed that the large volume change associated with the re- controlled stress conditions and interpreted them as a pattern action could generate high local stresses and strains, which of secondary porosity bands formed by dissolution coupled would cause episodic cracking. This idea has then been ap- to locally intensified compressional stresses. plied to olivine carbonation by Kelemen and Matter (2008), Previous investigations of olivine carbonation were largely who proposed a positive feedback loop where fractures could based on the interpretation of naturally deformed samples be generated during the volume-expanding reaction, porosity (e.g., Macdonald and Fyfe, 1985), thermodynamic modeling and permeability can be maintained or even increased, which (e.g., Kelemen and Hirth, 2012) or comparison with reac- in turn would accelerate the carbonation processes (Rudge et tion systems other than olivine (e.g., leucite to analcime in al., 2010). In 2011, Kelemen et al.
Details
-
File Typepdf
-
Upload Time-
-
Content LanguagesEnglish
-
Upload UserAnonymous/Not logged-in
-
File Pages18 Page
-
File Size-