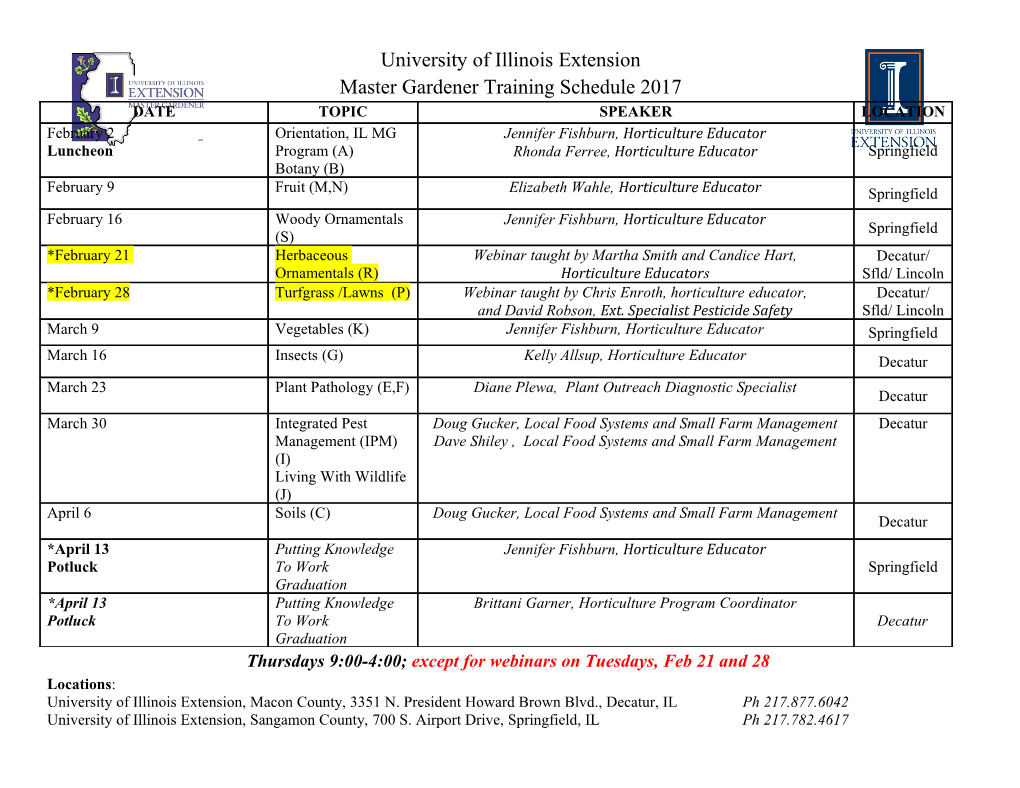
FACULTE DES SCIENCES Institut de Chimie Thérapeutique Assessment of drug permeation: Theory, methods and applications to skin and bacteria. Thèse de doctorat présentée à la Faculté des Sciences de l’Université de Lausanne par Sandrine Geinoz Pharmacienne diplômée de la Confédération Helvétique Université de Lausanne Jury Prof. François Marillier, Président Prof. Bernard Testa, Directeur de thèse Dr. Pierre-Alain Carrupt, Expert Prof. Richard Guy, Expert Dr. Patrizia Crivori, Expert Dr. Michel Neuwels, Expert LAUSANNE 2002 I Table of contents TABLE OF CONTENTS I LIST OF ABBREVIATIONS VII PART I: SKIN PERMEATION: A REVIEW 1 1 SKIN BARRIER FUNCTION 3 1.1 Introduction 3 1.2 The stratum corneum and routes of passive permeation 4 1.3 Characterization of the passive transdermal route of polar/ionic permeants 8 1.3.1 A dual-pathway model 8 1.3.2 A domain mosaic model 11 1.4 Factors influencing percutaneous absorption 15 1.4.1 Physiological factors 15 1.4.2 Extrinsic factors 18 1.5 References 20 2 BIOLOGICAL AND ARTIFICIAL MODELS TO STUDY SKIN PERMEATION 25 2.1 In vivo models 25 2.1.1 A pharmacokinetic approach to in vivo studies 25 2.1.2 Skin permeation in humans 26 2.1.3 Animal skin permeation 29 2.1.4 “Hybrid” models 30 2.2 In vitro models 31 II 2.2.1 Diffusion cells for measuring in vitro permeation 31 2.2.2 Skin models 32 2.2.3 Cell-culture techniques 35 2.2.4 Artificial membranes 36 2.3 Aims of the thesis 38 2.4 References 39 3 QUANTITATIVE STRUCTURE-PERMEATION RELATIONSHIPS (QSPERS) TO PREDICT SKIN PERMEATION: A CRITICAL REVIEW 47 3.1 Introduction 47 3.2 Statistical analysis 48 3.3 Common molecular parameters used in QSPeRs 48 3.3.1 Molecular weight and size 48 3.3.2 Solvatochromic parameters 51 3.3.3 Lipophilicity and related parameters 51 3.4 Quantitative structure-permeation relationships: pre-1990 period 53 3.5 Analyses based on Flynn’s dataset 54 3.6 Other recent QSPeR analyses 63 3.7 Conclusion 66 3.8 References 67 PART II: EXPERIMENTAL STUDIES ON SKIN PERMEATION 73 4 QUANTIFICATION OF LIPOPHILICITY 75 4.1 Experimental measurements of lipophilicity 75 4.1.1 Introduction 75 4.1.2 The shake-flask method 76 4.1.3 The potentiometric method 76 4.1.4 Cyclic voltammetry at the interface between two immiscible electrolyte solutions (ITIES) 78 III 4.2 Computational estimation of lipophilicity 83 4.3 References 85 5 SILICONE MEMBRANE: A MODEL FOR THE BARRIER FUNCTION OF SKIN 87 5.1 Introduction 87 5.2 Mathematical model 88 5.3 Materials and methods 90 5.3.1 Materials 90 5.3.2 pKa measurements 91 5.3.3 Partition coefficients measurements 91 5.3.4 Assessment of hydrogen-bonding capacity 92 5.3.5 Permeation experiments 92 5.3.6 Adjustment for ionization 94 5.4 Results and discussion 94 5.4.1 Permeability of phenolic compounds across silicone membranes 96 5.4.2 Permeability of selected drugs across silicone membranes 100 5.4.3 Comparison with human skin permeation 103 5.5 Conclusion 104 5.6 Appendix: Development of the mathematical model 105 5.7 References 108 6 MOLECULAR FIELDS IN QUANTITATIVE STRUCTURE- PERMEATION RELATIONSHIPS: THE VOLSURF APPROACH 111 6.1 Introduction 111 6.2 TLSER: a 2D-QSAR theoretical tool 111 6.3 Molecular fields: 3D-QSAR theoretical tools 112 6.3.1 The GRID force field 113 6.3.2 The Molecular Lipophilicity Potential (MLP) 114 6.3.3 The Molecular Hydrogen-Bonding Potential (MHBP) 115 6.4 The VolSurf procedure 119 IV 6.4.1 The water probe of GRID 120 6.4.2 The amide and carbonyl probe of GRID 120 6.4.3 The DRY probe of GRID 120 6.4.4 VolSurf and the 1D descriptors 122 6.5 Chemometrics tools 128 6.5.1 Principal Component Analysis (PCA) 128 6.5.2 Principal Component Regression (PCR) 130 6.5.3 Partial Least Squares (PLS) 130 6.5.4 Cross-validation 132 6.6 Application of 2D and 3D-QSAR analysis to skin permeation 133 6.6.1 Data validation criteria 134 6.6.2 Material and methods 141 6.6.3 Results and discussion 142 6.6.4 Conclusion 163 6.7 References 165 PART III: PARTITIONING OF IONS: INFLUENCE ON THE PERMEATION OF A SERIES OF SULFONAMIDES INTO BACTERIA 171 7 SULFONAMIDES AND BACTERIA PERMEATION 173 7.1 Classification and identification of bacteria 173 7.1.1 Bacteria: a definition 173 7.1.2 Taxonomy 173 7.2 Antibacterial sulfonamides 175 7.2.1 Historical background 175 7.2.2 Chemotherapeutic agents 176 7.2.3 Mechanism of action 177 7.2.4 Therapeutic use 179 7.2.5 Nomenclature and Classification 180 7.2.6 Structure and biological activity of sulfonamides 181 7.3 Materials and methods 183 V 7.3.1 Chemical compounds and reagents 183 7.3.2 Bacterial strain 184 7.3.3 Determination of the minimal inhibitory concentration (MIC) 185 7.3.4 pKa measurements 187 7.3.5 Partition coefficients measurements 189 7.3.6 Cyclic voltammetry measurements 189 7.4 Results and discussion 191 7.4.1 Viability of the microorganisms 191 7.4.2 Antibacterial activity of sulfonamides 196 7.4.3 Experimental versus theoretical lipophilicity 201 7.5 Conclusion 203 7.6 References 204 CONCLUSION 209 8 GENERAL CONCLUSION AND PERSPECTIVES 211 SUMMARY 215 RÉSUMÉ 216 V II List of abbreviations α, β Scaled values of H-bond donor and acceptor capacity π∗ Solvatochromic parameter standing for the solute's dipolarity/polarizability Σfac-(ihb) Sum of fragmental values (fβ) for H-bond acceptor capacity (taking into account intramolecular interactions) Σfdo-(ihb) Sum of fragmental values (fα) for H-bond donor capacity (taking into account intramolecular interactions) Σpi Sum of fragmental π∗ values alk n-alkane ATCC American type culture collection, catalog of reference strains CFU Colony forming units, number of bacterial colonies counted on a solid medium. dce 1,2-dichloroethane DM Diffusion coefficient in membrane M F Fischer's test GOLPE Generating optimal linear PLS estimations h Diffusional path length Kp Permeability coefficient L Membrane thickness log PI Logarithm of the partition coefficient of the ionic form log PN Logarithm of the partition coefficient of the neutral form log Pdce Logarithm of the partition coefficient measured in the 1,2-dichloroethane/water system log Poct Logarithm of the partition coefficient measured in the octanol/water system 0,I log Pdce Logarithm of the standard partition coefficient of the ionic form of an ionizable solute in the 1,2-dichloroethane/water system VIII ∆log P Difference between the log P values of a solute present in a single electric state, measured in two distinct solvent systems diff( logPN-I ) Difference between the log P values of the neutral and the ionized form of a given solute, measured in the same solvent system LSERs Linear solvation energy relationships LV Latent variable MHB Mueller-Hinton broth used as culture medium for bacterial growth MHBPs Molecular hydrogen-bonding potentials MHBPac(do) Acceptor (donor) MHBP MIC Minimal inhibitory concentration of an antibacterial agent to stop bacterial growth MIFs Molecular interaction fields MLP Molecular lipophilicity potential MLPhi(ho) Hydrophilic (hydrophobic) part of the MLP MxPs MHBPs or MLP fields oct n-octanol PCA Principal component analysis PC Principal component PLS Partial least squares q2 predictive correlation coefficient QSARs Quantitative structure-activity relationships QSPeRs Quantitative structure-permeation relationships r2 Square correlation coefficient s Standard deviation SC Stratum corneum SDEP Standard deviation of error of predictions TLSERs Theoretical linear solvation energy relationships VD Volume of the diffusion cell donor compartment VR Volume of the diffusion cell receptor compartment Vw Van der Waals volume Part I: Skin permeation: a review 9 kg … 3.6 kg fat off, 18'000 cm2 ! 1 cm2 3 blood vessels, 10 hairs, 12 nerves, 15 sebaceous glands, 100 sweat glands, 92 cm of blood vessels, 360 cm of nerves, 3'000'000 cells 3 1 Skin barrier function 1.1 Introduction Skin, the major function of which is to compartmentalize and protect an animal organism from its environment, was originally considered an essentially impermeable barrier. However, sensitive techniques of blood and urine analysis showed that the skin represents a potential portal of entry through which many chemicals can gain access to the systemic circulation. Consequently, much effort is currently directed towards utilizing the skin as a non-invasive route for drug administration [1]. One of the main advantages of transdermal drug delivery compared to oral administration is the avoidance of variability associated with the gastrointestinal tract (effects of pH, motility, transit time and food intake) and first-pass metabolism. Although these advantages are similar to those associated with intravenous infusion, transdermal drug delivery is a safer and more convenient method of drug administration [2]. During skin absorption, drugs have to pass a complex multilayer structure before reaching the blood [3]. Structurally, the skin can be viewed as a series of layers, the three major divisions being epidermis, dermis and hypodermis or subcutis (Fig. 1.1). The hypodermis lies below the dermis and functions as a fat-storage layer; being below the vascular system, this layer is not relevant to percutaneous penetration. The dermis (2 mm) is essentially an acellular collagen-based connective tissue that supports the many blood vessels, lymphatic channels and nerves of the skin. The extensive microvasculature network found in the dermis represents the site of resorption for drugs absorbed across the epidermis; at this point, transdermally absorbed molecules gain entry into the systemic circulation and access to their central targets.
Details
-
File Typepdf
-
Upload Time-
-
Content LanguagesEnglish
-
Upload UserAnonymous/Not logged-in
-
File Pages225 Page
-
File Size-