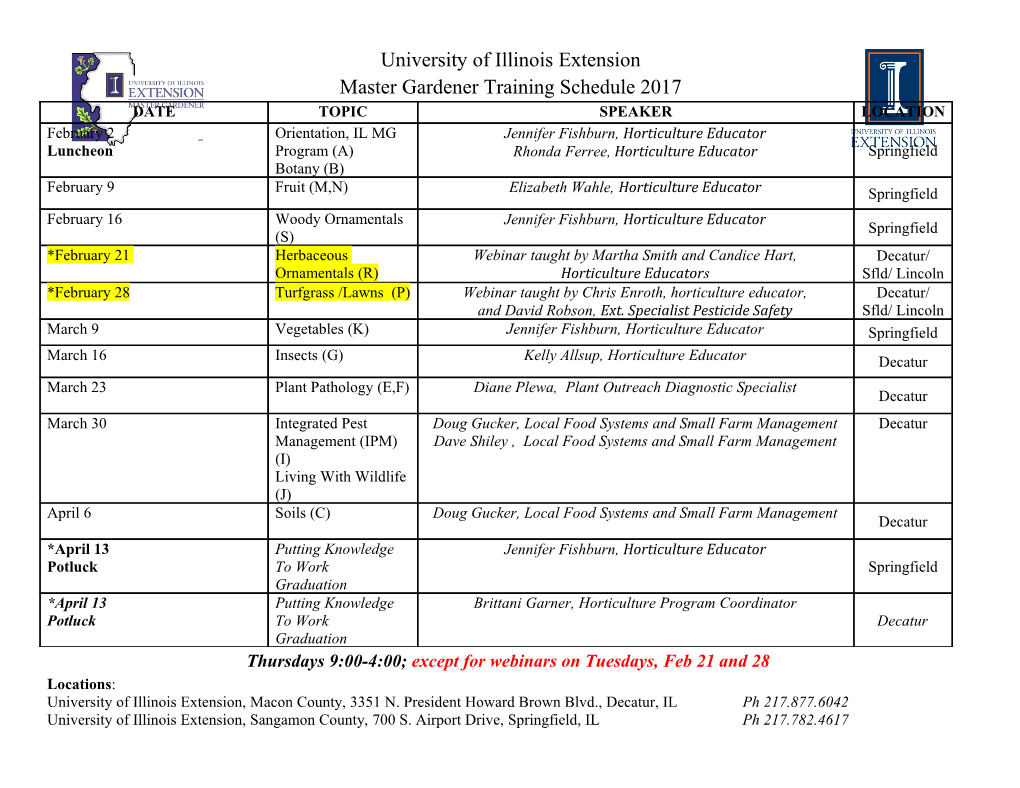
Ire1P 25 3 Ire1p: A Kinase and Site-Specific Endoribonuclease Tania N. Gonzalez and Peter Walter 1. Introduction 1.1. Ire1p in the Unfolded Protein Response The lumen of the endoplasmic reticulum (ER) is a highly specialized compartment in eukaryotic cells. Here, secretory and most membrane proteins are folded, covalently modified, and oligomerized with the assistance of specialized ER resident proteins (1). Perturbation of the ER lumen interferes with the production of many essential cellular components and can thus be highly deleterious. Indeed, in humans, defects in protein folding in the ER can lead to devastating diseases, such as cystic fibrosis, alpha1-anti- trypsin deficiency, and osteogenesis imperfecta (2). One way in which cells cope with the accumulation of unfolded proteins in the ER is by activating the unfolded protein response (UPR), an ER-to-nucleus signal transduction pathway (3–5). In the yeast Sac- charomyces cerevisiae, Ire1p is an essential component of this pathway. Ire1p is a transmembrane protein localized to ER membranes. When Ire1p was ini- tially identified as a component of the UPR, it was thought to function primarily as a serine/threonine kinase because of its homology to other kinases and its demonstrated kinase activity (6–8). Subsequent studies revealed that Ire1p functions as a site-spe- cific endoribonuclease as well (9). Both the kinase and endoribonuclease activities map to the carboxy-terminal half of Ire1p. The amino-terminal portion of Ire1p lies in the ER lumen (6,7), where it senses increases in the concentration of misfolded pro- teins. Since the ER and nuclear membranes are continuous, the carboxy-terminal portion of Ire1p is predicted to be located in either the cytoplasm and/or nucleus, where it induces downstream events in the UPR pathway. Immunoprecipitation of Ire1p from yeast-cell extracts has demonstrated that oligomerization of Ire1p coincides with its phosphorylation, suggesting that, as is the case for transmembrane receptor kinases, Ire1p can trans-autophosphorylate (10). Oligomerization of Ire1p coincides with induction of its endoribonuclease activity in vivo (9,10). The only cellular substrate known to be cleaved by Ire1p is the mRNA encoding the UPR-specific transcription factor, Hac1p. From: Methods in Molecular Biology, vol. 160: Nuclease Methods and Protocols Edited by: C. H. Schein © Humana Press Inc., Totowa, NJ 25 26 Gonzalez and Walter 1.2. Splicing of HAC1 mRNA by Ire1p and tRNA Ligase Hac1p upregulates transcription of genes encoding ER resident proteins by binding to a common regulatory sequence in their promoters (11–14). Cellular levels of Hac1p are controlled by the regulated splicing of its mRNA (13,15). In the absence of splicing, HAC1 mRNA translation is inhibited by a 252 nucleotide intron located at the 3'-end of the Hac1p coding region (15,16). Unlike the spliceosomal catalyzed splicing of all other pre-mRNAs known to date, removal of the HAC1 intron is catalyzed by the com- bined actions of Ire1p and tRNA ligase (9). When unfolded proteins accumulate in the ER, Ire1p initiates splicing by cleaving HAC1u mRNA (u for uninduced or unspliced) to liberate the intron and 5' and 3' exons. The exons are then joined by tRNA ligase, an enzyme previously thought to function exclusively in the splicing of pre-tRNAs. The ligated product, HAC1i mRNA (i for induced), goes on to be efficiently translated to produce Hac1p. Thus the regulated splicing of HAC1 mRNA is a key step in the UPR signaling pathway. The splicing of HAC1 mRNA by Ire1p and tRNA ligase is unprecedented. Signifi- cantly, Ire1p and tRNA ligase are sufficient to splice HAC1u mRNA in vitro (9,17). This is in striking contrast to the splicing of all other studied pre-mRNAs, which require the more than 100 proteins and small nuclear RNAs which constitute the spliceosome and its associated components (18). The splicing of HAC1u mRNA mechanistically resembles the splicing of pre-tRNAs (19,20). Ire1p and tRNA endonuclease both cleave to produce 5'-OH and 2',3'-cyclic phosphate RNA termini. Exon ligation by tRNA ligase in both splicing reactions follows the same chemical steps (17). Aside from these similarities, intriguingly, Ire1p and tRNA endonuclease are quite different. The two endoribonucleases lack any significant similarity in amino acid sequence or subunit composition, and recognize different structural features in their RNA substrates. Stem- loop structures predicted to form at the HAC1u mRNA splice junctions are required and sufficient to direct Ire1p cleavage. The stem-loop substrates that define the minimal Ire1p cleavage site are described elsewhere (17). 1.3. Ire1p Is Similar to RNase L The kinase and nuclease domains of Ire1p share significant homology with RNase L (8). RNase L cleaves RNA nonspecifically in cells infected with double-stranded RNA viruses, and has no known role in pre-mRNA processing (20,21). In vitro dimerization of RNase L induces its nuclease activity (23). Analogously, oligomerization of Ire1p correlates with its nuclease activity in vivo (9,10). In contrast to Ire1p, the kinase domain of RNase L appears to be catalytically inactive and has been shown to be involved in mediat- ing dimerization of the protein (23). The relationship between the kinase domain and nuclease activity of Ire1p has yet to be determined. We can follow the Ire1p kinase (9) and HAC1u RNA-splicing activities (9,17) in vitro by using a recombinant fragment carrying the Ire1p kinase and putative nuclease tail domains, Ire1p(k+t). The reconstituted splicing reaction has been used to define the minimal substrate requirements for Ire1p cleavage, determine the chemical nature of the RNA termini produced by Ire1p cleavage, and map Ire1p cleavage sites. Overall, we found that small stem-loop minisubstrate RNAs are sufficient to direct cleavage by Ire1p; that, like tRNA endonuclease, Ire1p endonucleolytic cleavage produces RNA Ire1P 27 fragments with 2',3'-cyclic phosphate and 5'-OH termini; and that Ire1p cleaves 3' of the guanosine residue found at each splice site (17). This chapter focuses on the meth- ods we have used during our studies of the kinase and nuclease activities of yeast Ire1p. 2. Materials 2.1. Equipment, Chemicals, and Molecular Biology Reagents 1. Equipment: Microfluidizer (Microfluidics, Newton, MA), scintillation counter. 2. Chemicals: Glycogen (Promega, Madison, WI), N-butanol, phenylmethylsulfonyl flouride (PMSF), tRNA, diethyl pyrocarbonate (DEPC), 10% Triton X-100, carbeni- cillin, isopropyl-1-thio-`-D-galactoside (IPTG), glutathione, LiCl, phenol, chloro- form, formamide. 3. Nucleotides: ADP, 5'pN, 5'pN2', 5'pN 3'p, and pN2'3'(cyclic)p (Sigma); [_-32P]NTP and [a-32P]ATP (3000 Ci/mM; Amersham, Arlington Heights, IL); NTPs; N = A, C, G, or U. 4. Enzymes: T7 RNA polymerase, nuclease P1, AMV reverse transcriptase, and mutant T4 polynucleotide kinase lacking 3' phosphatase activity (Roche Molecular Biochemicals); T4 polynucleotide kinase and SacI restriction enzyme (New England Biolabs, MA); PreScission Protease and ribonuclease U2 (Pharmacia, Uppsala, Sweden). 5. Miscellaneous: glutathione-Sepharose (Pharmacia, Uppsala, Sweden); RNasin ribonu- clease inhibitor (Promega, Madison, WI); Centricon-50 ultrafiltration units (Amicon, Beverly, MA); PEI cellulose thin-layer chromatography plates (EM Science, Gibbstown, NJ); X-ray film; Glogos II Autorad Markers (Stratagene, La Jolla, CA); plastic wrap (i.e., Saran Wrap), bovine serum albumin (BSA). 2.2. Solutions (see Note 1) 1. Crude extract buffer: 20 mM HEPES, pH 7.6, NaCl (0.35 or 1 M), 2 mM EDTA, 1 mM DTT, 10% glycerol, 1% Triton X-100. 2. 100 mM PMSF in ethanol. 3. Ire1p elution buffer: 20 mM HEPES, pH 7.6, 0.35 mM NaCl, 2 mM EDTA, 20 mM glu- tathione, pH 7.6. 4. Ire1p kinase buffer: 20 mM HEPES, pH 7.6, 250 mM K-acetate, 10 mM Mg(acetate)2, 1 mM DTT, 100 mM ATP. 5. Ire1p cleavage buffer: 20 mM HEPES, pH 7.6, 50 mM K-acetate, 1 mM Mg(acetate)2, 1 mM DTT, 2 mM ADP, 40 U RNasin. 6. PreScission protease buffer: 50 mM Tris-HCl, pH 7.0, 150 mM NaCl, 1 mM EDTA, 1 mM DTT, 10% glycerol. 7. Transcript elution buffer: 0.3 M Na-acetate, pH 5.2, 10 mM Mg(acetate)2. 8. Phenol/chloroform: mix equal parts phenol and chloroform. 9. TE: 10 mM Tris-HCl, pH 7.5, 1 mM EDTA, pH 8.0. 10. Stop solution: 50 mM Na-acetate, pH 5.2, 1 mM EDTA, 0.1% SDS. 11. RNA gel-loading buffer: 99% formamide, 1 mM Tris-HCl, pH 7.8, 0.1 mM EDTA, 0.1% SDS, xylene cyanol FF, Bromophenol blue. 12. Ribonuclease U2 buffer: 20 mM Na-citrate, pH 3.5, 1 mM EDTA, 7 M urea, 0.5 μg/μL tRNA. μ μ 13. Alkaline hydrolysis buffer: 50 mM NaHCO3, pH 9.0, 1 mM EDTA, 0.5 g/ L tRNA. 14. Nuclease P1 buffer: 20 mM Na-acetate, pH 5.2, 50 ng/μL tRNA. 15. 1 M LiCl. 16. Primer extension buffer: 20 mM NaCl, 15 mM HEPES, pH 7.6. 28 Gonzalez and Walter 2.3. Plasmids and Oligonucleotides 1. Vector for expression of recombinant S. cerevisiae Ire1p(k+t) in Escherichia coli: We expressed and purified a fragment of Ire1p fused at its amino-terminal end to glutathione- S-transferase (GST). This fragment (Ire1p amino acids 556–1115) carries the kinase (k) and putative nuclease tail (t) domains of Ire1p, and is termed GST-Ire1p(k+t). The expres- sion vector pCF210 (9) was made by subcloning the Ire1p(k+t) coding region into pGEX- 6p-2 (Pharmacia, Uppsala, Sweden). In this construct, a PreScission Protease cleavage site is encoded in the region that links GST and Ire1p(k+t) in the fusion protein. 2. HAC1U 508 RNA in vitro transcription vector: The vector pCF187 (9) was constructed by subcloning a PCR fragment carrying HAC1U mRNA sequences into pBluescript IISK(–) (Stratagene).
Details
-
File Typepdf
-
Upload Time-
-
Content LanguagesEnglish
-
Upload UserAnonymous/Not logged-in
-
File Pages12 Page
-
File Size-