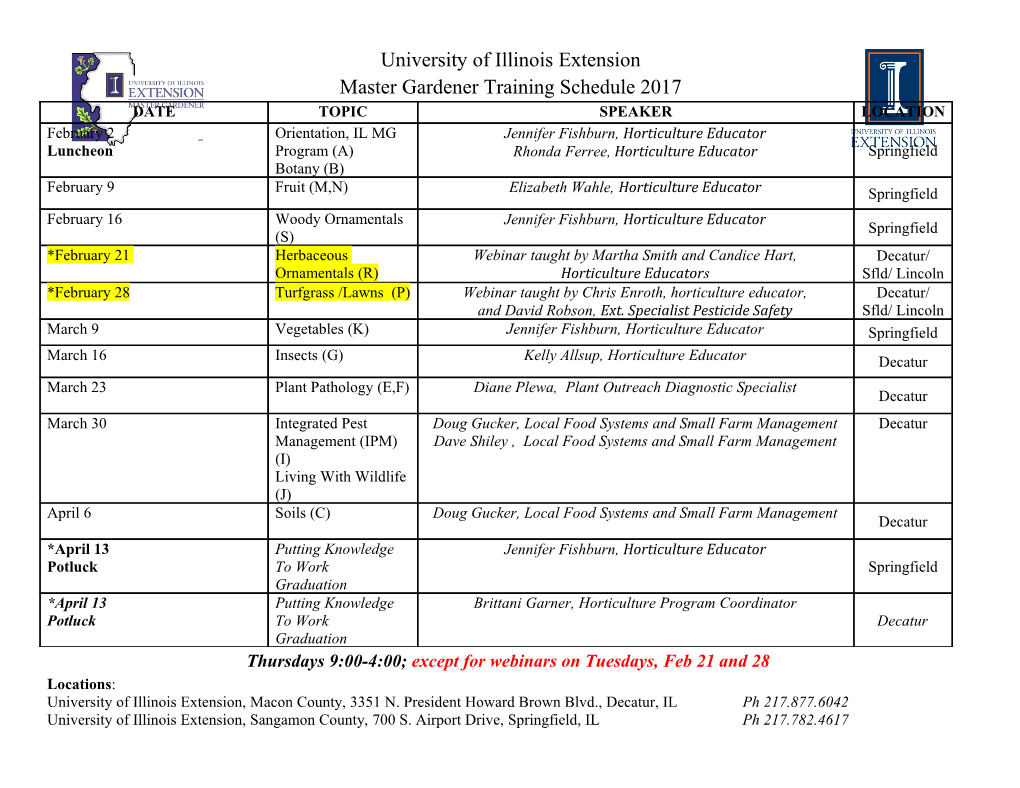
View metadata, citation and similar papers at core.ac.uk brought to you by CORE provided by Elsevier - Publisher Connector Redox Biology 4 (2015) 260–271 Contents lists available at ScienceDirect Redox Biology journal homepage: www.elsevier.com/locate/redox Review Article Interdependence of tetrapyrrole metabolism, the generation of oxidative stress and the mitigative oxidative stress response Andrea W.U. Busch a, Beronda L. Montgomery a,b,n a Department of Energy, Plant Research Laboratory, Michigan State University, 612 Wilson Road, East Lansing, MI 48824, USA b Department of Biochemistry and Molecular Biology, Michigan State University, 612 Wilson Road, East Lansing, MI 48824, USA article info abstract Article history: Tetrapyrroles are involved in light harvesting and light perception, electron-transfer reactions, and as co- Received 18 November 2014 factors for key enzymes and sensory proteins. Under conditions in which cells exhibit stress-induced Received in revised form imbalances of photosynthetic reactions, or light absorption exceeds the ability of the cell to use photo- 12 January 2015 excitation energy in synthesis reactions, redox imbalance can occur in photosynthetic cells. Such con- Accepted 14 January 2015 ditions can lead to the generation of reactive oxygen species (ROS) associated with alterations in tetra- Available online 16 January 2015 pyrrole homeostasis. ROS accumulation can result in cellular damage and detrimental effects on orga- Keywords: nismal fitness, or ROS molecules can serve as signals to induce a protective or damage-mitigating oxi- High light dative stress signaling response in cells. Induced oxidative stress responses include tetrapyrrole-de- Iron pendent and -independent mechanisms for mitigating ROS generation and/or accumulation. Thus, tet- Oxidative stress rapyrroles can be contributors to oxidative stress, but are also essential in the oxidative stress response to Photosynthesis fi ROS protect cells by contributing to detoxi cation of ROS. In this review, we highlight the interconnection and Tetrapyrrole interdependence of tetrapyrrole metabolism with the occurrence of oxidative stress and protective oxidative stress signaling responses in photosynthetic organisms. & 2015 The Authprs. Published by Elsevier B.V. This is an open access article under the CC BY-NC-ND license (http://creativecommons.org/licenses/by-nc-nd/4.0/). Contents Introduction............................................................................................................261 Light- and phytochrome-based responses to oxidative stress. 263 ROS as developmental signals . 263 Phytochrome-regulated oxidative stress response. 263 Photoreceptor-regulation of energy-dissipation mechanisms . 264 Tetrapyrroles and tetrapyrrole-containing proteins can mediate the cellular detoxification of reactive oxygen species . 264 Interconnection of oxidative stress, tetrapyrrole metabolism and nutrient availability. 264 Iron-induced oxidative stress . 265 Iron in the oxidative stress response . 265 Iron-tetrapyrrole interconnection. 265 Function of tetrapyrroles as signaling molecules and the oxidative stress response . 265 Tetrapyrroles, oxidative stress response and TspO . 266 Conclusions............................................................................................................266 Acknowledgements . 267 References.............................................................................................................267 n Correspondence to: Plant Research Lab, Michigan State University, DOE, 612 Wilson Road, East Lansing, MI 48824, USA. E-mail address: [email protected] (B.L. Montgomery). http://dx.doi.org/10.1016/j.redox.2015.01.010 2213-2317/& 2015 The Authprs. Published by Elsevier B.V. This is an open access article under the CC BY-NC-ND license (http://creativecommons.org/licenses/by-nc-nd/4.0/). A.W.U. Busch, B.L. Montgomery / Redox Biology 4 (2015) 260–271 261 Introduction Tetrapyrroles are linear or cyclic molecules containing four pyrrole rings that are ubiquitously utilized as cofactors in all kingdoms. These molecules are involved in central metabolic processes, including respiration, methanogenesis and photo- synthesis (reviewed in [1]). The most versatile tetrapyrrole co- factor is the porphyrin heme. This cyclic tetrapyrrole contains a central iron atom and is a major player in many cellular processes. Heme-bound proteins, i.e., hemoproteins, are involved in diverse functions ranging from oxygen transport to cellular signaling, energy transduction, lipid biosynthesis, and gene regulation, among others (reviewed in [1–3]). In photosynthesis, chlorophyll and the open-chain phycobilins are the most abundant and func- tionally important tetrapyrroles. Chlorophyll is present in the core photosystems of oxygenic photosynthetic organisms and the ex- tended light-harvesting complexes of plants and algae (reviewed in [4–6]). Phycobilins are found in the light-harvesting phycobili- somes attached to the core photosystems of algae and cyano- bacteria and phycobilins and related linear tetraypyrrole bilins serve as the chromophores of plant and bacterial photoreceptors (reviewed in [7]). Tetrapyrrole biosynthesis has been studied in great detail and is outlined in many excellent reviews [1,8]. A central portion of tet- rapyrrole synthesis yields products for chlorophyll synthesis and for production of heme and heme-derived tetrapyrroles, all of which are critical for photosynthesis and respiration. This part of tetrapyrrole synthesis includes the formation of protoporphyrin IX, a cyclic tetrapyrrole, after which the pathway bifurcates into the magnesium-dependent chlorophyll or iron-dependent heme branches (Fig. 1). The cleavage of heme and subsequent reduction of the biliverdin product yields bilins, which can act as chromo- phores for light-sensing photoreceptors or light-harvesting phy- cobiliproteins as introduced above. In this regard, tetrapyrroles are of special interest in the photosynthetic cell. Photooxidative stress is a core part of a photosynthetic lifestyle. It can be caused by overreduction of the photosynthetic apparatus when light absorbed exceeds the needs for carbon accumulation or the capacity for electron transfer. When light is in excess, en- ergy transfer from photoexcited chlorophyll in photosystem II to 1 oxygen results in singlet oxygen ( O2) formation [9–11]. Other reactive oxygen species (ROS) form through distinct mechanisms, e.g., electron transfer from electron acceptors of photosystem I to oxygen instead of ferredoxin primarily leads to superoxide anion À radical (O2 ) production [12]. The relatively stable ROS hydrogen peroxide (H2O2) can be produced due to reduction of superoxide, and can be detoxified by catalases or peroxidases (reviewed in [13,14])(note: see Table 1 for description of these and other mo- lecules involved in oxidative stress). However, H2O2 formation can also be catalyzed by metals such as iron to generate hydroxyl ra- dical (HO ) in Fenton chemistry [15]. Besides chlorophyll, other tetrapyrroles can act as photo- sensitizers due to their ability to absorb light of different wave- lengths. These molecules, thus, also pose a threat to the cell when they accumulate in their free form due to changes in their synthesis and utilization [16,17]. Central to cellular survival and Fig. 1. Tetrapyrrole biosynthesis. Eight molecules of δ-aminolaevulinic acid (ð-ALA) productivity, therefore, is a need to mitigate any potential damage form the tetrapyrrole ring. The cyclic tetrapyrrole protoporphyrin IX, a porphyrin, 2þ associated with the accumulation of free tetrapyrroles. Notably, an feeds into the magnesium-dependent (Mg ) chlorophyll or iron-dependent (Fe2þ ) heme pathway. The chlorophylls are essential components in the photo- accumulation of tetrapyrroles in the cell, e.g., by a change in flow systems, whereas the phycobilins serve in light-harvesting in the phycobilisome through biosynthetic pathways, leads to increased production and/ antennae [1]. or activity of ROS-detoxifying enzymes, including superoxide dis- mutase (SOD) and catalase enzymes [18]. Additionally, detox- causing damage to cells can support ROS scavenging [20]. These ification of redox-active heme can be achieved by heme-binding observations highlight the complex relationship between tetra- proteins through export, sequestration and/or degradation of pyrrole metabolism and oxidative stress. In this review, we pri- heme (reviewed in [19]). On the contrary, some tetrapyrroles have marily focus on the interdependence of tetrapyrroles and oxidative been reported to have antioxidant properties and thus rather than stress in photosynthetic organisms. 262 Table 1 Enzymes, molecules and protein complexes involved in oxidative stress and/or oxidative stress responses in photosynthetic organisms. Molecule Class Primary function Molecule/cofactor/ligand Role in oxidative stress Reference(s) Catalase Enzyme Decomposition of hydrogen peroxide Heme ROS detoxification For review see [177] Peroxidase Enzyme Decomposition of hydrogen peroxide Heme ROS detoxification For review see [177] Superoxide dismutase Enzyme Dismutation of superoxide radical Iron ROS detoxification For review see [177] into molecular oxygen (O2) or hydro- A.W.U. Busch, B.L. Montgomery / Redox Biology 4 (2015) 260 gen peroxide (H2O2) Rubrerythrin Enzyme Hydrogen peroxide reduction Iron Oxidative stress defense [111] N/Aa Light-absorbing, Cofactors, chromophores, etc. Tetrapyrroles, porphyrins (e.g., Photosensitizers, antioxidants,
Details
-
File Typepdf
-
Upload Time-
-
Content LanguagesEnglish
-
Upload UserAnonymous/Not logged-in
-
File Pages12 Page
-
File Size-