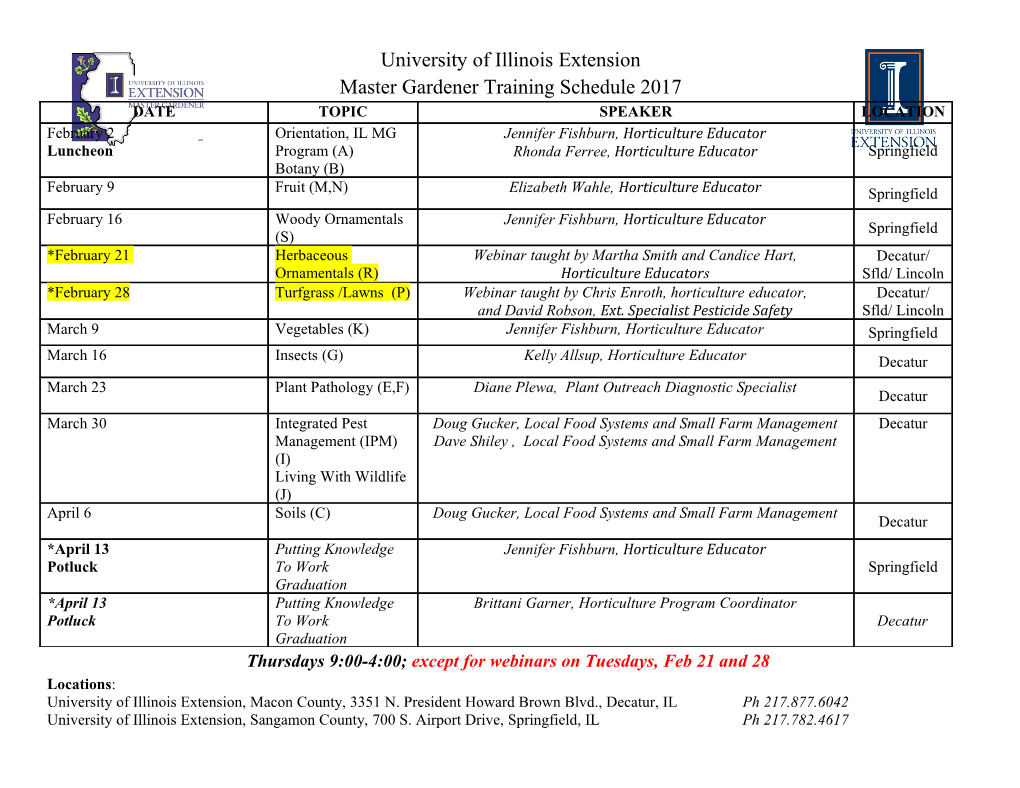
TRNT _^______—J WIS-91/74/Oct.-PH Ultrafast Secondary Emission 2D X-ray Imaging Detectors A. Akkerman,1 A. Breskin, R. Chechik,2 V. Elkind, I. FVumkin3 and I. Gibrekhterman The Weizmann Institute of Science 76100 Rehovot, Israel Presented at the European Workshop on X-ray Detectors for Synchrotron Radiation Sources Aussois, France, September 30-October 4, 1991 Also Soreq Research Center, Yavne, Israel The Hettie H. Heinemann Research Fellow Technion. Haifa, Israel Ultra fast Secondary Emission 2D X-ray Imaging Detectors A. Akkerman,1 A. Breskin, R. Chechik,2 V. Elkind, I. Frumkin3 and I. Gibrekhterman The Weizmann Institute of Science 76100 Rehovot, Israel Abstract We describe a new method for subnanosecond, parallax-tree, high accuracy X-ray imaging at high photon flux. X-rays are ronverted in thin Csl layers. Secondary emitted electrons are amplified in multistage electron multipliers, operating at low gas pressures (10-40 Torr). Introduction Fast and accurate large area X-ray imaging is requested in many applications in basic and applied re­ search. Examples are particle identification with Transition Radiation, plasma diagnostics, medical or indus­ trial radiography and X-ray diffraction experiments with high luminosity Synchrotron Radiation Sources. Gaseous wire chambers, traditionally used for X-ray imaging1' over a large area, are often too slow (100 ns typical resolution) and suffer from parallax errors in localization, due to different photon absorption depths in the detection volume. Space charge effects limit the counting rate of traditional gaseous detectors. Re­ cently developed gaseous ji-strip detectors2* are also based on photon conversion in the gas, and therefore suffer from the same limitations. Other X-ray detectors', mostly 6olid state devices or MCP's, as reviewed by Arndt3*, may have good time and localization characteristics but are limited in size. A different approach is to convert the photons in a thin solid layer and to detect the secondary electrons emitted from the impact point. The great advantage in using solid convertors is the fact that very good local­ ization and timing properties may be achiefrdd when amplifying the low-energy secondary emitted electrons at their production location. High quantum yields for soft X-rays are reported, for example, by Fraser et al.4* using Csl X-ray convertors coated on MCP secondary electron multipliers. Such devices are mostly used in astrophysics. Margarian5* proposed to couple solid, gold convertors to gaseous wire chambers filled with helium at at­ mospheric pressure for the localization of Transition Radiation photons. A similar idea was proposed by Do- rion et al.6* for industrial radiography with high energy X-rays, using tantalum convertors. The yield of secondary electrons from solid convertors depends on the X-ray energy, and on the convertor material and thickness. High detection efficiencies can be reached at low grazing angles, where the "'effective" thickness of the convertor is high while the escape thickness for the secondary electron is low5'. The most ef­ ficient convertor for soft X-rays, often used in MCP X-ray detectors4*, seems to be Csl, which is very efficient in converting the few KeV energy of the primary photoelectron to a large size cloud of low energy (<50 eV) secondary electrons. A detailed discussion is given by Henke et al.7* and by Schwarz8*. The combination of solid convertors and ii.&hly efficient low pressure gaseous electron multipliers was proposed by Breskin9*. The idea was to couple UV-sensitive photocathodes, or Csl convertors for relativistic particle detection, to a low-pressure MWPC9* or multistage10' electron multiplier. The high amplification factors (> 10T) reached in these electron multipliers10-12) allow for high detection efficiency even of single electrons. Their high rate capability is derived from reduced space charge effects, due to fast ion removal and to a low charge density in the electron avalanche. The latter also reduces aging effects. The fast amplification mechanism leads to a subnanosecond time resolution10*. Single electrons can be localized with an accuracy of the order of 200 /im FWHM10*. Secondary emission X-ray detectors based on a Csl convertor, coupled to low-pressure gaseous electron multipliers were proposed by Majewski13*. First results of such devices were reported by Breskin et al.14*. A detailed discussion of the method, its advantages and its possible application to particle identification by Transition Radiation Detection (TRD) is given by Akkerman et al.15*. The detector The secondary emission X-ray detector is shown in fig 1. A convertor foil is coupled to a multistage avalanche electron multiplier, operating at low gas pressure. Our prototype detector consists of several, 80 mm diameter, mesh and wire electrodes on G-10 frames, mounted in a vacuum vessel. A thin window, made of 100 pm Kapton, was used. The photocathode of 400- Also Soreq Research Center, Yavne, Israel The Hettie H. Heinemann Research Fellow Technion, Haifa, Israel - 1 - I 80D0 A Csl, is vacuum deposited on 200 A of Al to provide the potential. The suhstrati* is 2 5 /JCII thick my­ lar The electrons emitted from the convertor are immediately amplified in a :j mm wid-> parallel gap The avalanche electrons are transferred directly, or through a transfer gap (not shown in tin* lig ). and further am­ plified m a MWPC element, .'quipped with cathode deJay-Jjne readout. A deiajjed description of this element and the readout system is given elsewhere16'. The data acquisition and analysis is done with a PC computer interfaced to CAM AC based electronics ^ADC's TDC's). The detector was operated with 10-10 Torr of ethane, isobulane or dimethylether (DME). These gases provide high gain, up to lu' for single electrons, even in a single parallel gap The detector was tested wiih ti-tiO keV X-rays, at normal incidence and at a grazing angle of ")° (tf=s$5°). lu addition to the higher conver­ sion efficiency, the grazing angle geometry also has improved localization resolution in the projected dimen­ sion, by a factor of cosO (see fig. 1). PHOTOCATUCDE CN SUPPORTING PLASTIC fClL / PAHALZLL PLAT- ' PREAMPLIFIER / 'Win \ N WIRE GRID i \ MWPC /. .1 schematic view of the Secondary Emission X-ray detector. A solid comer/or is coupled to a low-pressure W-=<?E=3uRE jASCIO-sO^lfifi mulhstep secondary electron multiplier. Experimental results Figure 2 shows pulse height spectra obtained from a 2000 A thick Csl convenor bombarded with a) 8 keV X-rays and b) with L'V photons. One can notice the large amplitude avalanches from X-ray induced sec­ ondary electrons, as compared to the single UV-pUotoelectron. spectrum. Due to the high efficiency of the electron multiplier, the total X-ray detection efficiency is almost identical to the X-ray conversion efficiency. Figure 3 shows our calculated values of conversion efficiency in a 2000 A thick Csl layer. This is. according to Henke et al.'', the optimal convenor thickness, allowing the efficient escape of the low energy secondary electrons. For example. 6 keV X-rays are converted with an efficiency of about 6% at normal incidence. The efficiency increases to about 30% at a grazing angle of 10°. The detection efficiency was measured at 8 keV for various Csl convertor thickness. The best result of t=3.5(/c was obtained at 3000 A. The efficiency measured with a 2000 A convertor, is in reasonable agreement with the calculated value of fig. 3. The detection efficiency at 8 keV was also measured at a grazing angle of 5°. The measured value is of the order of 22% as compared to the 35% calculated efficiency. Part of the discrepancy may originate from some error in the determination of the angle of incidence and from other ge­ ometrical limitations of the experimental setup, It should be noted that the pulse height spectra obtained at normal incidence and at 5° have the same shape, as shown in fig. 2a. a) b) 2. The pulse-height distribution of secondary electrons induced by a) 8 keV photons, b) UV-photons. in a '2000 A thick Csl convenor. Isobutane. 18 Torr. The rednced electric field in the preampUjicatwn gap is 180 Y/cmTorr. 4. The time response of the detector to about twenty simultaneous photoelectrons. Peak separation is 4 ns. the FWHM is 350 ps, CH* at 10 Torr. 3. The conversion efficiency of X-ray photon in a 2000 A thick Csl layer, for a normal En 9rg LksV] incidence and a grazing angle of\Q°. 1 The time resolution of the detector was measured with a pulsed UV-photon source, of which the pho­ ton yield could be tuned17'. Figure 4 slfcMs a time distribution measured with light pulses inducing approx­ imately 20 phocoelectrons, the FYV1TM is 350 ps. The time resolution is of the order of 0.35 - -Ins (FW1IM), for 20 to 1 emitted secondary photoelectrons respectively. It should be noted that the full area of the detec­ tor was used for extracting the timing signals (6-1 cm2). The time resolution could be greatly improved if the electrode is subdivided into small readout sections. Time resolutions of a few hundreds of ps (FWHM) arc therefore expected with Csl, since it yields on the average 17 secondary electrons per converted X-ray photon8'. The localization properties of our prototype detector were investigated with collimated X-rays, mostly with Csl photocathodes. Al and Au convectors did not provide satisfactory ,esults, due to their higher yields of primary (energetic) electrons''14'. The intrinsic resolution with 8 keV X-rays, at normal incidence, is bet­ ter than 200 fim (FWHM). As discussed above, a much better resolution is obtained at a grazing angle of 5°, shown in fig.
Details
-
File Typepdf
-
Upload Time-
-
Content LanguagesEnglish
-
Upload UserAnonymous/Not logged-in
-
File Pages5 Page
-
File Size-