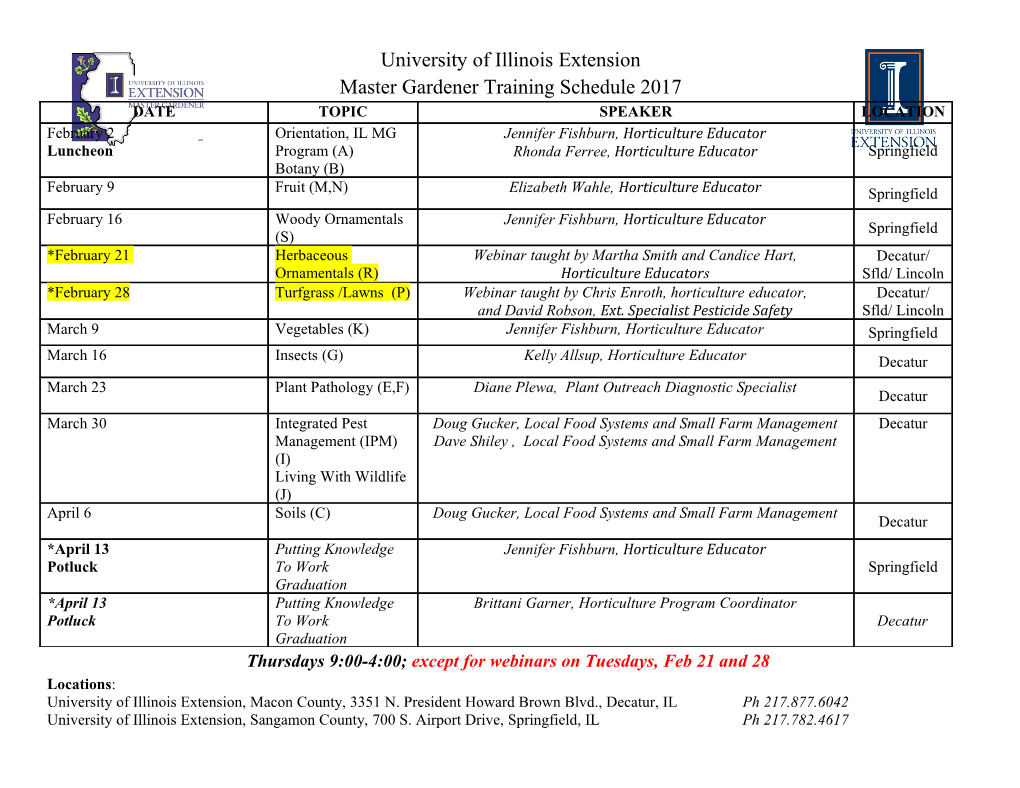
SOME OXIDATION REACTIONS OF SUBSTITUTED AMIDES A thesis presented by ROGER MUNRO UPTON in partial fulfilment of the requirements for the degree of DOCTOR OF PHILOSOPHY •of the UNIVERSITY OF LONDON Imperial College London June 1978 2 Abstract Recent transition metal-catalysed reactions of peroxides, of preparative value in organic synthesis, have been reviewed. Molybdenum, vanadium, and tungsten compounds typically promote electrophilic oxidations, notably epoxidation and oxidation at nitrogen and sulphur, often involving metal-directed regio- and stereoselecti- vities. A second category of catalysed peroxide reactions involves the formation of hydroxyl, alkoxy, and acyloxy radicals from, for example, iron and copper-catalysed decompositions of various organic peroxides. Secondary amides and heteroaromatic amides have been converted into the corresponding hydroxamic acids by the oxidation of the trimethylsilylamide derivatives using molybdenum pentoxide complexes. Primary amides, ureas, urethanes, and imides were generally inert towards similar oxidation conditions. The oxidation of N-silylated Leterocyclic compounc::. by molybdenum pentoxide (Mo0 5.L) gave the N-oxides of benzimidazole, adenine, and 4-quinolone. The i3C n.m.r. spectra of the parent amides, the ethyl imidates, and the trimethylsilylamides of series of para-substituted acetanilides, aliphatic lactams, and heteroaromatic amides are reported and the concentrations of the trimethylsilylamide tautomers evaluated by inte- gration of their 13C n.m.r. spectra. The trapping of a postulated 1,3-dipole [_c4j,+ generated from diaziridinones, N-chloroureas, -semicarba- zides, and -methoxyureas, and from N-carbamylsulphilimines has been unsuccessfully attempted. Alternative reaction 3 pathways gave an imidazoline from dimethylurea, 1-methyl- -4-phenyl-1,2,4-triazolidin-3-one from dimethylsemicarba- zide, and 3,3- dimethyl-4-phenyl-1,2,4-oxazolidin-5-one from hydroxyphenylurea. Furthermore, a silver(I) catalysed conversion of N-chloromethoxyureas into aryl or alkyl urethanes via formation of the free isocyanate was ob- served. 4 Contents Abstract 2,3 List of Tables 5 Acknowledgements 6 Part I : Review 1. Some transition metal-catalysed reactions of 8 peroxides: A. Electrophilic reactions 9 B. Radical reactions 43 References to Part I 62 Part II : Results and Discussion 2. The synthesis of hydroxamic acids by the 71 oxidation of trimethylsilylamides 3. 13C N.m.r. studies of trimethylsilylamides 110 4. Oxidation reactions of molybdenum pentoxide 128 5. Reactions of activated ureas 135 Experimental 155 References to Part II 200 5 List of Tables 1. Silylation and oxidation results for secon- 82 dary amides. 2. Data for silylation of p-substituted 84 acetan ilides. 3. Oxidation data for AVO-trimethylsilylanilides 85 4. Silylation and oxidation data for aryl 88 carboxamides. 5. Silylation and oxidation data for multifunc- 90 tional secondary amides. 6. Silylation and oxidation data for heteroaro- 95 matic amides. 7. Silylation data for ureas, urethanes, and 96 imides. 8. Data from 'H n.m.r. spectra of trimethyl- 103 silylacetanilide. 9. Data from I H n.m.r. spectra of trimethylsilyl- 103 -2-pyrrolidone. 10. 13C Chemical shifts of acetanilide deriva- 116 tives. 11.C Chemical shifts of p-chloroacetanilide 117 derivatives. 12.C Chemical shifts of p-methoxyacetanilide 118 derivatives. 13.C Chemical shifts of p-nitroacetanilide 119 derivatives. 14. Substituent effects on the shielding of 120 aromatic carbon nuclei. 15. 13C Chemical shifts of 2-pyrrolidone deriva- 122 tives. 16.C Chemical shifts of E-caprolactam deriva- 122 tives. 17. 1 3C Chemical shifts of 2-pyridonederivatives. 123 18. t3C Chemical shifts of 4-quinolone and 4- 124 -pyridone derivatives. 19. Concentrations of silylamide tautomers in 126 13C n.m.r. spectra. 20. Silylation and oxidation of nitrogen hetero- 130 cycles. 6 Acknowledgements The author wishes to express his sincere appreci- ation to Professor P.G. Sammes, for his enthusiastic encouragement and advice during the course of this work. The author is indebted to Shell for providing a C.A.S.E. award and, in particular, to Dr. R.J.G. Searle for his supervision during the work carried out at the Shell Laboratories, Sittingbourne. The author wishes to thank the technical staff at Imperial College --Mr. K.I. Jones for microanalyses, Mrs. Lee for mass spectra, and especially Mr. Jack Peppercorn, Chris, and Dorothy --; the technical staff at the City University; and also Mrs. E. Baldeo for the typescript. Finally, thanks must go to Alan Weedon, Robert Watt, Nigel Walsh, Janet Markham, Lynn Davies, Nigel Broom, Guido Serra-Errante, All Jaxa-Chamiec, and other colleagues who helped with discussions and provided a friendly atmosphere in which to work. 7 PART I REVIEW 8 CHAPTER 1 SOME TRANSITION METAL-CATALYSED REACTIONS OF PEROXIDES An extensive body of literature exists on the action of metal ions upon peroxides. This covers a wide range of topics, many of which are of significant in- dustrial value, and which include the use of peroxides, catalysed by metals, as polymerisation initiators, 2 in autoxidation, and in organic syntheses,3 and the inorganic aspects of metal catalysis, particularly in relation to biological processes.4 This introductory review covers recent aspects of the oxidations of organic substrates by peroxides in the presence of transition metals. Two general classes of metal-catalysed peroxide oxidations have been distinguished. Low-valent transition elements, typically iron (II) and copper (I), catalyse the generation of free radicals and these oxidations are characterised by the redox reactions of radical species with metal ions. 5 The second category comprises reactions catalysed by, for example, molybdenum (VI), vanadium (V) 9 and tungsten (VI) compounds, which form metal-peroxide complexes that promote polar, electrophilic oxidations. A. Electrophilic Reactions The earliest examples of electrophilic oxidations relate to the formation of glycols from olefins by the reaction of hydrogen peroxide catalysed by vanadium pentoxide s or tungstic acid .7 The intermediate epoxides were also made accessible with, for example, tungsten trioxide and, later, by the use of organic hydroperoxides, catalysed by soluble metal complexes. 9,10 A general affinity for heteroatoms,11 hydroxylations at carbon and nitrogen, dehydrogenations and the ketolisation of olefins have also been observed. As such, transition metal- catalysed oxidations with hydroperoxides bear resemblance to the reactions of peroxycarboxylic acids and yet these reagents show valuable differences in regio- and stereo- selectivities .12''3 Superior catalytic activity has been attributed to a high degree of Lewis acidity and low oxidation potential of the metal. 14,15 These properties appear to be most effectively combined in 1to(VI), V(V), W(VI), and Ti(IV)16 though many other metals exhibit some catalytic activity.17 Homogeneous or heterogeneous systems can be used, and recently, polymer-supported catalysts18 have demonstrated improved reactivities. However, substitution-labile catalysts are required to permit coordination with the hydroperoxides (cf. Scheme 1); thus the substitution -inert complexes of EDTA and diethylenetriamine are in- effective catalysts for epoxidation. 19 Stable metal- 10 peroxo complexes (8) 20 have also been used in organic synthesis and these provide useful additions to the metal- catalysed hydroperoxide oxidations. 1. Epoxidation Owing to its industrial potential the epoxidation of olefins by the catalysis of tert-alkylhydroperoxides has been mainly investigated. 21a Molybdenum compounds appear to be the best homogeneous or heterogeneous catalysts,10 consistently achieving very high epoxide yields over a wide range of conditions. An exception occurs in the epoxidation of allylic alcohols for which vanadium com- pounds are superior to molybdenum. The scope of catalysts, hydroperoxides, and other reaction parameters have been summarised in earlier reviews.21 Mechanism - Metal-Hydroperoxide Complexes The mechanism of epoxidation of olefins using hydro- peroxides in the presence of molybdenum or vanadium catalysts is generally considered to involve an electrp- philic oxidant, 21a demonstrated by the negative correlation between the epoxidation rate of m and p-substituted styrenes and the Taft substituent parameters.22° Competi- tive free radical decomposition of the hydroperoxide also occurs .23 Many groups have examined the kinetics of metal- catalysed epoxidations,1°, 16, 22- 25 and apparent first- order relationships with respect to the hydroperoxide, the olefin substrate, and homogeneous catalysts have been observed. Recent studies 15,22 have established the validi- ty of rate equations that further incorporate the alcohol 11 by-products, which are strong inhibitors of the reaction. These observed kinetics closely relate to the Michaelis- Menton equations for enzyme catalysis with product inhi- bition. The generally accepted mechanism is outlined in Scheme 1, for vanadium catalysts. 25 Similarly, molyb- denum-catalysed reactions involve metal-hydroperoxide intermediates, 15,22 although the roles of molybdenum- olefin complexes 26 and molybdenum-peroxo complexes,2? analogous to the epoxidations by molybdenum pentoxide (Scheme 5), have also been speculated. Scheme 1 V n+ activation vv [1] + RO2H V!--OR [2] I (1) OH V-11-- R + + H0~ [3] OH V!--OR + 0`I [4] H V!-OR + RO2H Vv--OR + ROH [51 H OH ROH ROH Vv V1-(ROH) --- V- (ROH)2 [6] 12 An initial, rapid oxidation of the
Details
-
File Typepdf
-
Upload Time-
-
Content LanguagesEnglish
-
Upload UserAnonymous/Not logged-in
-
File Pages209 Page
-
File Size-