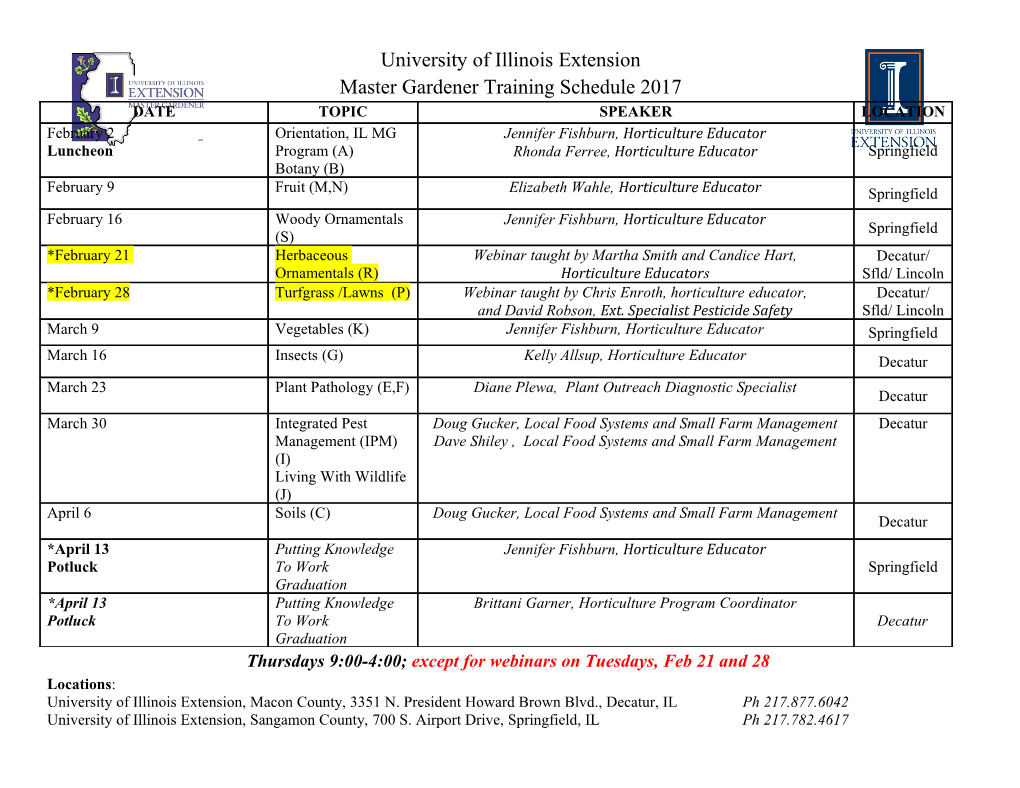
Thandapani et al., p 1 1 2 Aven recognition of RNA G-quadruplexes regulates 3 translation of the Mixed Lineage Leukemia protooncogenes 4 5 Palaniraja Thandapani1,2,3,4‡, Jingwen Song1,2,3,4‡, Valentina Gandin1, Yutian Cai1,5, Samuel G. 6 Rouleau6, Jean-Michel Garant6, Francois-Michel Boisvert7, Zhenbao Yu1,2,3,4, Jean-Pierre 7 Perreault6, Ivan Topisirovic1,3,5, and Stéphane Richard1,2,3,4* 8 9 Running title: Aven regulates G4 motif mRNA translation 10 11 Keywords: Arginine methylation, PRMT1, mRNA translation, polysomes, Aven, RGG/RG 12 motif, G-quadruplexes, mixed lineage leukemia, acute lymphoblastic leukemia. 13 14 1Terry Fox Molecular Oncology Group and Segal Cancer Center, 2Bloomfield Center for 15 Research on Aging, Lady Davis Institute for Medical Research and 3Departments of Oncology, 16 4Medicine, and 5Biochemistry, McGill University, Montréal, Québec, Canada H3T 1E2. 17 6Département de Biochimie, 7Département d’Anatomie et de Biologie Cellulaire, Faculté de 18 Médecine et des Sciences de la Santé, Pavillon de Recherche Appliquée au Cancer, Université de 19 Sherbrooke, Québec, Canada J1E 4K8 20 ‡authors contributed equally 21 *Corresponding author: Lady Davis Institute, 3755 Côte Ste-Catherine Road, Montréal, Québec, 22 Canada H3T 1E2. Phone: (514) 340-8260, Fax: (514) 340-8295 23 E-mail: [email protected] 24 Thandapani et al., p 2 25 Abstract 26 G-quadruplexes (G4) are extremely stable secondary structures forming stacks of guanine 27 tetrads. DNA G4 structures have been extensively studied, however, less is known about G4 28 motifs in mRNAs, especially in their coding sequences. Herein, we show that Aven stimulates 29 the mRNA translation of the mixed lineage leukemia (MLL) proto-oncogene in an arginine 30 methylation-dependent manner. The Aven RGG/RG motif bound G4 structures within the coding 31 regions of the MLL1 and MLL4 mRNAs increasing their polysomal association and translation, 32 resulting in the induction of transcription of leukemic genes. The DHX36 RNA helicase 33 associated with the Aven complex and was required for optimal translation of G4 mRNAs. 34 Depletion of Aven led to a decrease in synthesis of MLL1 and MLL4 proteins resulting in 35 reduced proliferation of leukemic cells. These findings identify an Aven-centered complex that 36 stimulates the translation of G4 harboring mRNAs, thereby promoting survival of leukemic cells. 37 38 Thandapani et al., p 3 39 Introduction 40 RNA binding proteins (RBPs) coordinate many steps of RNA metabolism ranging from splicing, 41 RNA processing, RNA transport, mRNA translation and RNA degradation (Glisovic et al., 42 2008). RBPs associate with specific RNA motifs and/or secondary structures within coding, 43 untranslated regions and non-coding RNAs in functional units called ribonucleoprotein (RNP) 44 complexes (Mitchell and Parker, 2014). Defects in RBPs have been associated with many 45 complex diseases ranging from neurological disorders to cancer (Castello et al., 2013; Cooper et 46 al., 2009; Lukong et al., 2008; Ramaswami et al., 2013). 47 RBPs are predominantly defined by the presence of RNA binding domains within their 48 sequences (Chen and Varani, 2013). Recently, several ‘interactome capture’ strategies have been 49 performed to identify RBPs genome-wide. In addition to identifying the known RBPs, these 50 approaches have identified numerous mammalian proteins that do not possess a canonical RNA 51 binding domain (Baltz et al., 2012; Castello et al., 2012; Kwon et al., 2013). Interestingly, RBPs 52 that harbor repeated sequences including YGG and RGG motifs were identified (Castello et al., 53 2012). The RGG/RG motif is enriched in proteins associated with RNA and is a known RNA 54 binding interface (reviewed in (Thandapani et al., 2013)). The RGG/RG motif, also called RGG 55 box, was shown to bind RNA (Kiledjian and Dreyfuss, 1992). Subsequently, the RGG/RG motifs 56 of Nucleolin, FMRP, FUS and EWS were also shown to bind guanine-rich sequences that are 57 potential G-quadruplexes (Darnell et al., 2001; Haeusler et al., 2014; Takahama et al., 2011; 58 Takahama et al., 2013). RGG/RG motifs also mediate protein-protein interactions. Notably, the 59 RGG/RG motif of yeast Scd6 mediates interactions with eIF-4G which leads to stress granule 60 formation and inhibition of cap-dependent translation (Rajyaguru et al., 2012). Despite these Thandapani et al., p 4 61 recent advances, little is known about the role of RGG/RG motifs that bind both RNA and 62 proteins. 63 G-quadruplexes (G4) are planar structures of stacks of guanine tetrads stabilized by monovalent 64 potassium or sodium ions. G-quadruplexes have been shown to regulate DNA replication, DNA 65 repair, gene expression and telomeres (Bates et al., 2007). Less is known about G4 structures 66 found in RNA. There are > 1500 potential G4s (PG4s) in 5′-UTR of mRNAs alone (Beaudoin 67 and Perreault, 2010), but not all PG4s form stable G-quadruplexes, which are influenced by the 68 numbers of G-quartets, the possibility of bulge formation, the length of the loops and the 69 presence of alternative Watson-Crick base pair-based stable structure (Burge et al., 2006; Jodoin 70 et al., 2014; Mukundan and Phan, 2013). Some PG4s in the 5′-UTR of mRNAs form bona fide 71 G-quadruplexes and inhibit cap-dependent translation (Beaudoin and Perreault, 2010; Bugaut 72 and Balasubramanian, 2012; Kumari et al., 2007). Recently, inhibitors of DEAD box RNA 73 helicase eIF4A, or eIF4A1 depletion have been shown to selectively inhibit translation of 74 mRNAs with G-quadruplexes in their 5’UTR (Modelska et al., 2015; Wolfe et al., 2014). 75 However, presence of G-quadruplexes in 5’UTRs does not appear to be sufficient to render 76 translation of mRNAs sensitive to changes in eIF4A activity (Rubio et al., 2014). In addition to 77 the incomplete understanding of the role of 5’UTR G-quadruplexes in translation control, little is 78 known about how G4 structures in open reading frames (ORFs) affect translation. 79 Arginine residues within RGG/RG motifs are preferred substrates for methylation by protein 80 arginine methyltransferases (PRMTs) (Thandapani et al., 2013). Arginine methylation is known 81 to regulate many cellular processes including signal transduction, transcription, pre-mRNA 82 splicing, and DNA repair (Bedford and Clarke, 2009; Bedford and Richard, 2005; Xu et al., 83 2013). PRMT1 generates > 85% of asymmetric dimethylarginines found in cells with preference Thandapani et al., p 5 84 for RGG/RG motif containing proteins (Bedford and Clarke, 2009). PRMT1 is known for its 85 nuclear roles in regulating gene expression and DNA damage (An et al., 2004; Boisvert et al., 86 2005; Strahl et al., 2001; Wang et al., 2001), however less is known about its cytoplasmic roles. 87 PRMT1-deficient mice die at E6.5 and the absolute removal of PRMT1 in mouse embryo 88 fibroblasts (MEFs) leads to cell death (Pawlak et al., 2000; Yu et al., 2009). To identify other 89 biological processes regulated by arginine methylation, we performed a bioinformatics approach 90 to identify proteins harboring RGG/RG motifs and one such protein we identified was Aven 91 (Thandapani et al., 2013). 92 Aven is a predominantly cytoplasmic protein required for cell survival and it has been shown to 93 function as an apoptotic inhibitor by interaction with and stabilizing the pro-survival protein Bcl- 94 xL, as well as inhibiting the function of Apaf-1 (Chau et al., 2000). It was proposed that the 95 proteolytic cleavage of Aven by Cathepsin D is required for its anti-apoptotic activity (Melzer et 96 al., 2012). Furthermore, Aven is required for ataxia telangiectasia mutated (ATM) activation in 97 Xenopus oocytes and HeLa cells (Guo et al., 2008) and ataxia telangiectasia related (ATR) 98 activation following DNA damage in osteosarcoma cells (Baranski et al., 2015). High Aven 99 expression correlates with poor survival in metastatic patients with osteosarcomas (Baranski et 100 al., 2015). The elevated Aven expression is also frequently observed in acute myeloid leukemia 101 (AML) and acute lymphoblastic leukemia (T-ALL) and is associated with poor prognosis (Choi 102 et al., 2006; Paydas et al., 2003). A transgenic mouse model with T cell-specific overexpression 103 of Aven showed that its expression enhanced T-cell lymphomagenesis in the absence of p53 104 (Eismann et al., 2013). The mechanism by which Aven promotes hematological malignancies is 105 yet to be understood. Thandapani et al., p 6 106 Herein, we report that the methylation of the RGG/RG motif of Aven functions in the 107 translational control of mRNAs harboring G4 structures in their ORFs. The association of Aven 108 with polysomes was dependent on the arginine methylation of its RGG/RG motif and on the 109 methyl-dependent interactions with the Tudor domains of SMN and TDRD3, previously shown 110 to be associated with polysomes (Goulet et al., 2008; Sanchez et al., 2013). We identify Aven to 111 be an RNA binding protein, as its RGG/RG motif bound G4 motifs in the ORFs of mRNAs 112 encoding the mixed lineage leukemia (MLL) family proteins MLL1 and MLL4. The RGG/RG 113 motif of Aven also associated with the G4 RNA helicase, DHX36, and this helicase was required 114 for optimal translation of Aven regulated mRNAs. Furthermore, Aven deficient T-ALL cell lines 115 had reduced MLL1 and MLL4 protein levels, but not mRNA levels, which were paralleled by 116 proliferation defects. These findings define a hitherto unknown mechanism of action for arginine 117 methylation in regulating translation of a subset of mRNAs including those encoding pivotal 118 leukemogenic transcriptional regulators MLL1 and MLL4. 119 Thandapani et al., p 7 120 Results 121 Aven RGG/RG motif is methylated by PRMT1 122 Aven harbors an N-terminal RGG/RG motif (Thandapani et al., 2013), a nuclear export sequence 123 (NES) (Esmaili et al., 2010) and a predicted BH3 domain (Hawley et al., 2012) (Figure 1A).
Details
-
File Typepdf
-
Upload Time-
-
Content LanguagesEnglish
-
Upload UserAnonymous/Not logged-in
-
File Pages72 Page
-
File Size-