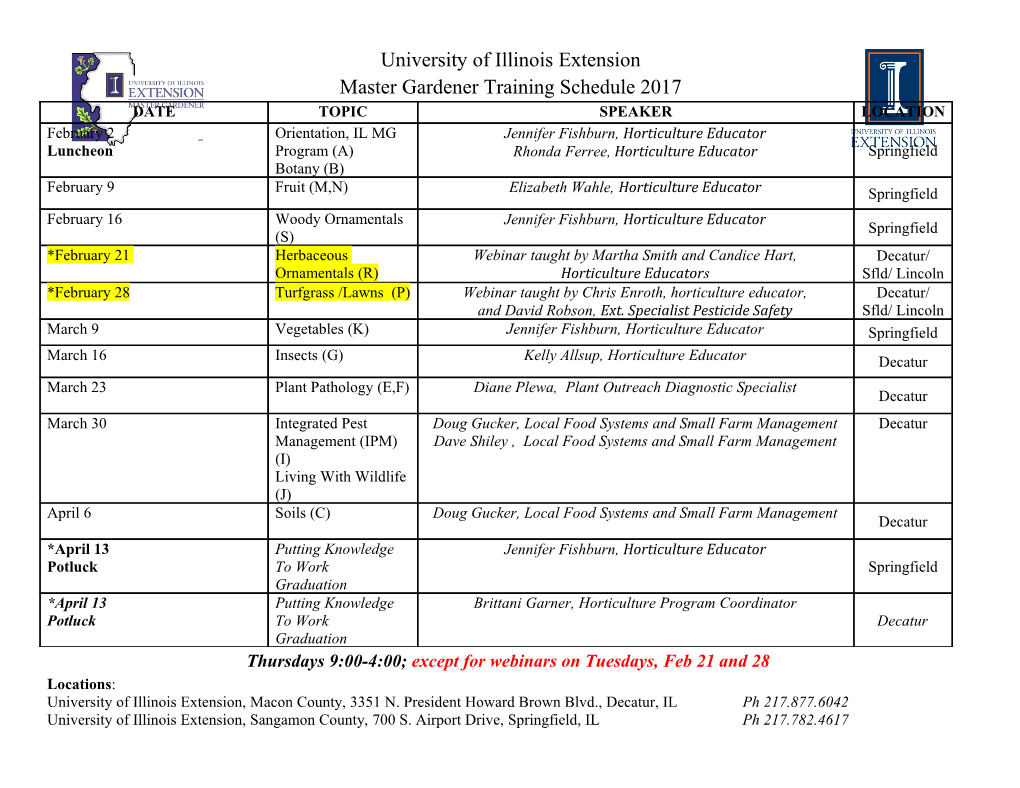
NIH Public Access Author Manuscript Free Radic Biol Med. Author manuscript; available in PMC 2014 March 01. NIH-PA Author ManuscriptPublished NIH-PA Author Manuscript in final edited NIH-PA Author Manuscript form as: Free Radic Biol Med. 2013 March ; 56: 89–101. doi:10.1016/j.freeradbiomed.2012.11.010. Aldehyde Dehydrogenases in Cellular Responses to Oxidative/ electrophilic Stress Surendra Singh1, Chad Brocker1, Vindhya Koppaka1, Chen Ying1, Brian Jackson1, Akiko Matsumoto2, David C. Thompson3, and Vasilis Vasiliou1,* 1Department of Pharmaceutical Sciences, Skaggs School of Pharmacy and Pharmaceutical Sciences, University of Colorado Anschutz Medical Campus, Aurora, CO 80045, USA 2Department of Social Medicine, Saga University School of Medicine, Saga 849-8501, Japan 3Department of Clinical Pharmacy, Skaggs School of Pharmacy and Pharmaceutical Sciences, University of Colorado Anschutz Medical Campus, Aurora, CO 80045, USA Abstract Reactive oxygen species (ROS) are continuously generated within living systems and the inability to manage ROS load leads to elevated oxidative stress and cell damage. Oxidative stress is coupled to the oxidative degradation of lipid membranes, also known as lipid peroxidation. This process generates over 200 types of aldehydes, many of which are highly reactive and toxic. Aldehyde dehydrogenases (ALDHs) metabolize endogenous and exogenous aldehydes and thereby mitigate oxidative/electrophilic stress in prokaryotic and eukaryotic organisms. ALDHs are found throughout the evolutionary gamut, from single celled organisms to complex multicellular species. Not surprisingly, many ALDHs in evolutionarily distant, and seemingly unrelated, species perform similar functions, including protection against a variety of environmental stressors like dehydration and ultraviolet radiation. The ability to act as an ‘aldehyde scavenger’ during lipid peroxidation is another ostensibly universal ALDH function found across species. Up-regulation of ALDHs is a stress response in bacteria (environmental and chemical stress), plants (dehydration, salinity and oxidative stress), yeast (ethanol exposure and oxidative stress), Caenorhabditis elegans (lipid peroxidation) and mammals (oxidative stress and lipid peroxidation). Recent studies have also identified ALDH activity as an important feature of cancer stem cells. In these cells, ALDH expression helps abrogate oxidative stress and imparts resistance against chemotherapeutic agents such as oxazaphosphorine, taxane and platinum drugs. The ALDH superfamily represents a fundamentally important class of enzymes that significantly contributes to the management of electrophilic/oxidative stress within living systems. Mutations in various ALDHs are associated with a variety of pathological conditions in humans, underscoring the fundamental importance of these enzymes in physiological and pathological processes. Keywords Aldehyde dehydrogenase; Cancer stem cells; Chemical stress; Dehydration; Electrophilic stress; Oxidative stress © 2012 Elsevier Inc. All rights reserved. *Corresponding author: Vasilis Vasiliou, Ph.D., [email protected], phone: 1 (303) 724-3520, fax: 1 (303) 724-7266. Publisher©s Disclaimer: This is a PDF file of an unedited manuscript that has been accepted for publication. As a service to our customers we are providing this early version of the manuscript. The manuscript will undergo copyediting, typesetting, and review of the resulting proof before it is published in its final citable form. Please note that during the production process errors may be discovered which could affect the content, and all legal disclaimers that apply to the journal pertain. The authors have no conflicts of interest related to the work detailed within this manuscript. Singh et al. Page 2 Introduction NIH-PA Author Manuscript NIH-PA Author Manuscript NIH-PA Author Manuscript Aldehyde dehydrogenases (ALDHs) are involved in a variety of biological processes in prokaryotic and eukaryotic organisms. Their expression is up-regulated in response to abiotic and biotic stress generated by perturbed endobiotic and/or xenobiotic metabolism. Such stress-responsive expression of ALDHs manifests in a broad range of plant and animal species, underscoring the evolutionary conservation of biological adaptions to oxidative and electrophilic stresses (Table 1). Living organisms are constantly confronted by oxidative stress and the reactive oxygen species (ROS) derived therefrom. In animals, inflammation, mitochondrial respiration, xenobiotic metabolism and other processes generate oxidants that contribute to ROS formation. ALDHs are known to decrease oxidative stress, particularly that caused by aldehydes [1, 2]. ALDH induction has been observed in a variety of plants species exposed to heat, dehydration, salinity, oxidants, ultraviolet (UV) radiation, pesticides or metals. Mechanical trauma and fungal infection can also elicit ALDH up-regulation in plants [3]. Pathogenic bacteria encounter oxidative stress emanating from the host immune response that must be overcome during invasion and sustained infection [4, 5]. Organisms, including yeast and Caenorhabditis elegans, also express a variety of ALDHs in response to oxidative stress [6, 7]. The representation of the ALDH gene superfamily in all three taxonomic domains (Archaea, Eubacteria and Eukarya) is suggestive of a crucial role for these enzymes throughout evolutionary history [8]. Aldehydes are strongly electrophilic, highly reactive and relatively long-lived compounds. Reactive aldehydes readily form adducts with DNA, RNA and proteins, leading to impaired cellular homeostasis, enzyme inactivation, DNA damage and cell death [9-11]. They have been implicated in oxidative stress-associated diseases, such as atherosclerosis, cancer, diabetes, chronic alcohol exposure, acute lung injury, and in neurodegenerative diseases like Alzheimer’s and Parkinson’s disease [9, 12, 13]. The ALDH superfamily contains NAD(P)+-dependent enzymes that oxidize a wide range of endogenous and exogenous aldehydes to their corresponding carboxylic acids [1]. The ability of ALDHs to act as ‘aldehyde scavengers’ is grounded in the observation that many have broad substrate specificities and can metabolize a wide range of chemically- and structurally-diverse aldehydes. Many of the ALDH isozymes overlap in relation to substrate specificities, tissue distribution and subcellular localization but vary in their efficiency in metabolizing specific aldehydes [14-17]. The human genome contains 19 protein-coding ALDH genes. ALDH proteins are found in one or more subcellular compartments including the cytosol, mitochondria, endoplasmic reticulum and nucleus, as well as plastids in plants [14]. Mutations and polymorphisms in ALDH genes are associated with various pathophysiological conditions in humans and rodents [1, 18] including Sjögren-Larsson syndrome [19], type II hyperprolinemia [20], γ- hydroxybutyric aciduria [21], pyridoxine-dependent epilepsy [22], hyperammonemia [23], alcohol-related diseases [24], cancer [25] and late-onset Alzheimer’s disease [14, 26] (Table 2). ALDH enzymes may also play important antioxidant roles by producing NAD(P)H [27, 28], directly absorbing UV radiation [29, 30] and scavenging hydroxyl radicals via cysteine and methionine sulfhydryl groups [31]. Aldehyde generation and metabolism Aldehydes are generated during metabolism of various endobiotic and xenobiotic compounds. For example, aldehydes are associated with the metabolism of alcohols, amino acids (e.g., lysine, valine, proline and arginine), anticancer drugs (e.g., cyclophosphamide) and neurotransmitters (e.g., -aminobutyric acid (GABA), serotonin, noradrenaline, adrenaline, dopamine) [1, 32, 33]. Lipid peroxidation (LPO) of cellular phospholipids induces the formation of more than 200 highly-reactive aldehyde species, including 4- hydroxy 2-nonenal (4-HNE), malondialdehyde (MDA), 4-oxononenal (4-ONE), acrolein, Free Radic Biol Med. Author manuscript; available in PMC 2014 March 01. Singh et al. Page 3 crotonaldehyde and methylglyoxal [14, 34, 35]. Environmental pollutants, such as smog, cigarette smoke, motor vehicle exhaust, pesticides and various food additives, either contain NIH-PA Author Manuscript NIH-PA Author Manuscriptor contribute NIH-PA Author Manuscript to the formation of aldehydes, including formaldehyde, acetaldehyde and acrolein [14, 34, 35]. While some aldehydes play vital roles in normal physiological processes, including vision, embryonic development and neurotransmission, many aldehydes are cytotoxic and carcinogenic [14, 25]. ALDHs play critical roles in metabolizing these endogenous and exogenous molecules. In addition to acting as general aldehyde scavengers, many ALDHs play important roles in homeostatic pathways. Five members of the ALDH family, viz. ALDH1A1, ALDH1A2, ALDH1A3, ALDH1A7 and ALDH8A1, catalyze the irreversible conversion of retinaldehyde to retinoic acid (RA), which plays critical role in developmental process by modulating retinoid signaling [1, 36]. ALDH1L1, also known as 10-formyltetrahydrofolate dehydrogenase (FDH), is involved in the conversion of 10-formyltetrahydrofolate to tetrahydrofolate, a critical reaction for replenishing the cellular folate pool [1, 37]. Several ALDHs are also involved in the detoxification of LPO-derived reactive aldehydes, which are implicated in promoting covalent modification of proteins and DNA and in diseases resulting from such modifications [38, 39]. ALDH3A2 catalyzes the oxidation of fatty aldehydes;
Details
-
File Typepdf
-
Upload Time-
-
Content LanguagesEnglish
-
Upload UserAnonymous/Not logged-in
-
File Pages35 Page
-
File Size-