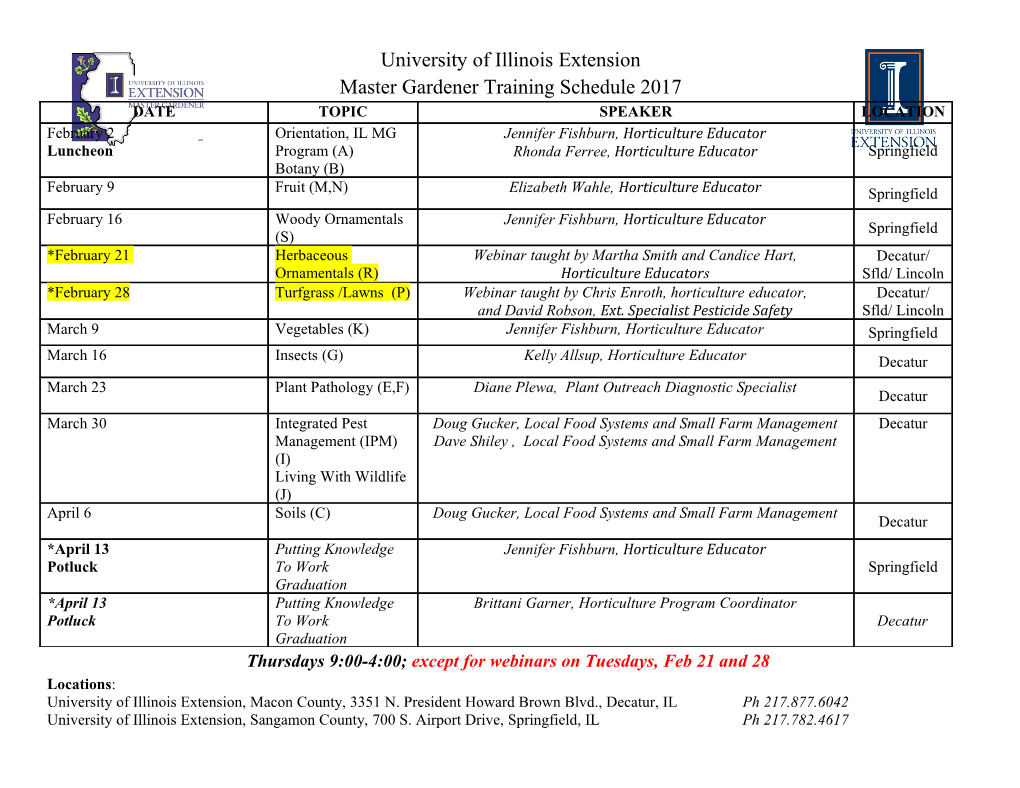
An Investigation into Carbon Flow through the Metabolic Networks of Rhodobacter sphaeroides DISSERTATION Presented in Partial Fulfillment of the Requirements for the Degree Doctor of Philosophy in the Graduate School of The Ohio State University By Michael Steven Carter Graduate Program in Microbiology The Ohio State University 2014 Dissertation Committee: Dr. Birgit Alber, Advisor Dr. F. Robert Tabita Dr. Joseph Krzycki Dr. Tina Henkin Copyright by Michael Steven Carter 2014 Abstract Predicting carbon flow within an organism requires a complete knowledge of the pathways that compose the organism’s metabolic networks. Even in pathways that have been biochemically characterized, an incomplete knowledge of the regulation of the responsible genes and respective enzymes disallows prediction of carbon flow through the entire network. Therefore, in order to predict the flow of carbon molecules through metabolic networks, the corresponding enzymes must be characterized and their regulation must be elucidated. Toward a better understanding of metabolic carbon flow, the following work investigated core metabolism in the purple nonsulfur bacterium Rhodobacter sphaeroides 2.4.1. As a member of the purple nonsulfur bacteria, R. sphaeroides is especially metabolically versatile, an indication of the diversity of pathways within its metabolic networks. By investigating the interplay of the pathways that compose the networks, this work intends to offer insights into the strategies employed by R. sphaeroides as it modulates carbon flow through its metabolic networks. Chapter 2 begins the investigation by examining which enzymes participate in carbon flow through the C4/C3 node. The C4/C3 node is responsible for converting metabolic intermediates between the citric acid cycle (C4) and glycolysis/gluconeogenesis (C3). The growth of a series of strains with mutations in ii genes of key enzymes of the node was examined across various carbon sources. Specifically, strains with mutations in two different genes that encode malic enzymes – responsible for catalyzing the oxidative decarboxylation of malate to pyruvate – were compromised in their ability to grow with some substrates that require C4 to C3 conversion, such as succinate and (S)-malate, but their growth was unimpaired during growth with acetate which is assimilated via succinate and malate. As an additional apparent contradiction, a pyruvate phosphate dikinase mutant was unable to grow on acetate, suggesting that gluconeogenesis occurs exclusively from pyruvate during acetate growth. While the mode of C4 to C3 conversion in R. sphaeroides remains an open question, growth results with a pyruvate carboxylase mutant indicated that pyruvate carboxylase is primarily responsible for C3 to C4 conversion on substrates that are predicted to be assimilated through C3 intermediates. Chapters 3 and 4 examine the role of carbon flow through intermediary pathways of short chain acyl-CoA assimilation in R. sphaeroides. R. sphaeroides employs the ethylmalonyl-CoA pathway for acetyl-CoA assimilation. The enzymes of the pathway are encoded by genes that are dispersed throughout the genome, and no previous information exists regarding strategies for their regulation. The pathway’s first committed reaction is catalyzed by crotonyl-CoA carboxylase/reductase (Ccr) and includes reactions that are shared with polyhydroxybutyrate biosynthesis. Transcript levels of ccr were observed to be 30-fold higher in extracts of acetate-grown cells than succinate-grown cells, and ccr promoter-reporter fusions were likewise regulated, demonstrating a transcriptional regulation of ccr expression. Mutating the gene that iii encodes PhaR, a transcriptional regulator of polyhydroxybutyrate synthesis, did not affect the observed regulation of Ccr activity. Regulation of propionyl-CoA assimilation was also characterized here. PccR was identified as a transcriptional activator and repressor of pccB, the gene for the β-subunit of propionyl-CoA carboxylase of the methylmalonyl-CoA pathway for propionyl-CoA assimilation. Transcript levels of pccB in wild type R. sphaeroides were 11-fold higher ̅ during propionate/HCO3 growth compared to levels during succinate growth. When pccR was mutated, pccB transcript levels and propionyl-CoA carboxylase activities were equivalent in succinate- and acetate-grown cells but higher than levels in wild type cells grown with succinate. Mutation analyses with pccB promoter-reporter fusions revealed the PccR operator site within the pccB promoter as two proximal TTTGCAAA motifs. Despite the presence of a single truncated palindrome (TTGCAA) upstream of ccr and the role of PccR in regulating the methylmalonyl-CoA pathway, a component of the ethylmalonyl-CoA pathway, ccr expression was unaffected by mutation of pccR. Based on homology to PccR, a family of regulators of assimilation of short chain fatty acyl-CoA molecules was identified (the ScfR family). The relative sequence similarity among the proteins further classifies them into four different classes – RamB, MccR, PccR, and IbcR. In nearly every case, genes for members of each class clustered with genes of the glyoxylate bypass (RamB), the methylcitrate cycle (MccR), the methylmalonyl-CoA pathway (PccR), or genes for the assimilation of isobutyryl-CoA (IbcR). Three hundred twenty-seven proteins were identified, and a comparison of the iv sequence immediately preceding their genes identified putative operator sequences for some of the classes. The sum of the evidence presented herein continues the characterization of modes by which carbon flows through metabolic networks. The work in Chapter 2 highlights the obscurity that remains regarding the control of carbon flow through the C4/C3 node despite the biochemical characterization of its enzymes. Chapter 3 similarly seeks to initiate an intense study of the regulation of a single control point in the cell’s assimilation of acetyl-CoA. Chapter 4 turns the perspective outward as it identifies a source of regulation of propionyl-CoA and extrapolates the use of the regulatory strategy throughout many bacteria. Together, the sum of the contribution of the chapters serves to clarify possible schemes for metabolic network control while emphasizing the necessity for further clarification. v Dedication Dedicated to my mom Ann Pendley, my wife Stacia Kock, and my best friend Einstein. The unyielding determination modelled by my mom was a constant source of motivation for the completion of the work presented in and the construction of this document. All of which was performed through the sanity provided by the smiles, laughs, and love from Stacia and Einstein. vi Acknowledgments My gratitude goes to Dr. Birgit Alber for the time that she committed to my training. The critical analysis that she provided through her unmatched attention to detail often allowed me to refine the precision of my reasoning and presentation of data. For their advice, I also thank my dissertation committee – Dr. F. R. Tabita, Dr. Joseph Krzycki, and Dr. Tina Henkin. Many of the experiments presented here were accomplished almost exclusively through the generosity and expertise of Dr. Tabita and the members of his laboratory. Specifically, I would like to thank Dr. Sriram Satagopan and Dr. Rick Laguna for advice on experimental and professional details. I am grateful for Dr. Krzycki’s ability to simultaneously explain scientific concepts while challenging me to fully incorporate their tenets. I also appreciate Dr. Henkin’s thoughtful professional advice, regular communication about career opportunities, and rigorous examination of my scientific assertions. As an unofficial member of my committee, Dr. Charles Daniels often made himself available to analyze and critique my conclusions based on my data. Within my laboratory, I am grateful to have worked with Dr. Marie Asao whose organizational techniques were a true model to the success that accompanies an organized mind. Also, I am glad to have worked with Dr. Kathrin Schneider who provided friendship and scientific conversation in my early graduate career. More currently, I vii appreciate the friendship offered by Steven Carlson. He often provided levity that brightened a sunless laboratory and an unfaltering but tolerant will that challenged my scientific understanding. I also appreciate the personal support of Dr. Lauren Branditz and Kelsey Baron who were undergraduate researchers in the laboratory at various times and were great friends to me. Much of my work builds upon the careful work that they completed during their time in the laboratory. Finally, I thank Dr. Jessica Spears and members of the Alfonzo laboratory. Dr. Spears provided an unparalleled combination of friendship and scientific discourse that was at the core of the growth that I experienced throughout graduate school. The other members of the Alfonzo laboratory, especially Dr. Juan Alfonzo and Dr. Mary Anne Rubio, offered unprecedented inclusiveness and joviality to improve the scientific lifestyle. viii Vita 2007...............................B.S. Microbiology, B.A. Biochemistry Indiana University Bloomington, IN 2007 to present ..............Graduate Teaching and Research Associate Department of Microbiology The Ohio State University Columbus, OH Publications Schneider, K., M. Asao, M. S. Carter, B. E. Alber. 2012 Rhodobacter sphaeroides uses a reductive route via propionyl coenzyme A to assimilate 3-hydroxypropionate. J. Bact. 194(2): 225. Fields of Study Major Field: Microbiology ix Table of Contents Abstract
Details
-
File Typepdf
-
Upload Time-
-
Content LanguagesEnglish
-
Upload UserAnonymous/Not logged-in
-
File Pages244 Page
-
File Size-