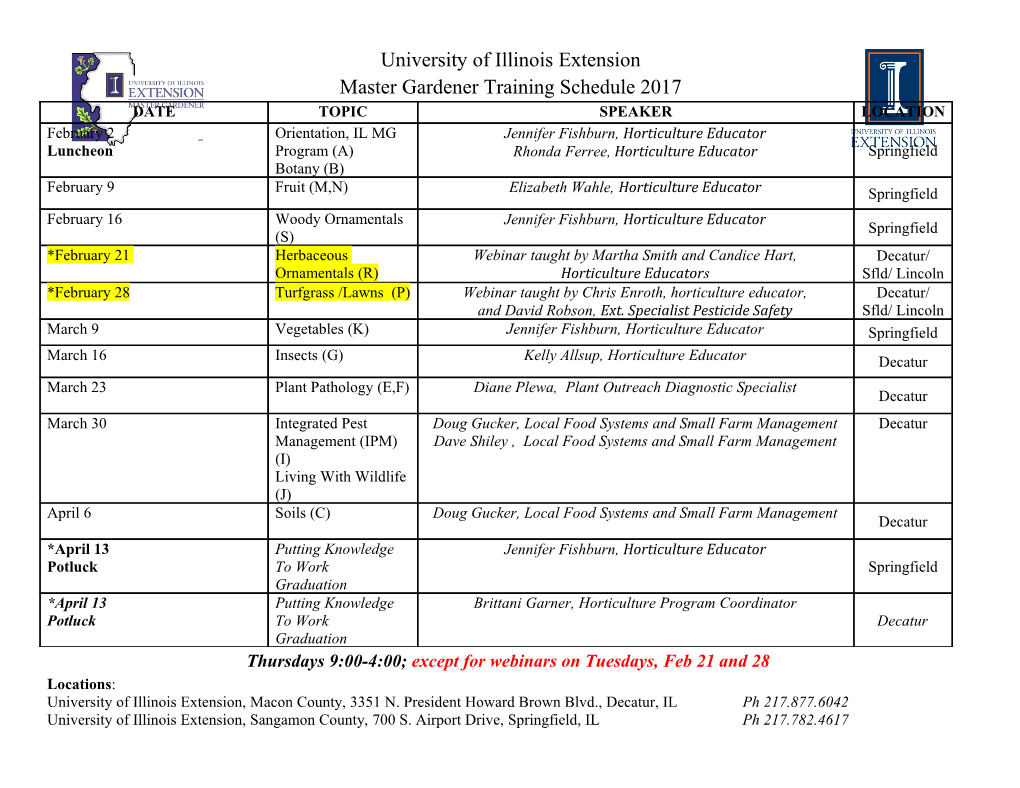
Appendix A How Can We Detect Radiation? If our tongues were as sensitive as these radiation detectors, we could easily taste one drop of vermouth in five carloads of gin. Dixy Lee Ray 1914–1994 It is impossible to smell, see, taste, hear or feel ionising radiation. Humans have no senses for α, β and γ rays, so detectors are needed, to play the part of this absent capability – to sense ionising radiation for us. It is necessary to measure radiation exposures so that they can be monitored, controlled and limited. Humans also have to be protected against unexpected exposures. One important duty is to supervise radiation-exposed workers, and to measure external radiation exposures, contami- nations and incorporations, particularly in working areas. Another is to protect the environment against unnecessary exposures. This includes the determination of radi- ation exposures of the general public, the monitoring of the disposal of radioactive waste into the environment, and the examination of the distribution of radioactive material in the biosphere (the atmosphere, soil, water, and eventually food). National radiation-protection authorities have realised that radiation exposures from natural sources also have to be considered. In certain situations, natural radiation can increase the radiation level for particular individuals in the population quite considerably. Therefore, these natural sources cannot be neglected in the framework of radiation protection. The detectors used in the field of radiation protection have to be reliable and robust, and their measurements have to be reproducible. It is important to note that for each of the various types of radiation, an appropriate detector needs to be used. This chapter introduces the various types of radiation detector normally used in industry, academia and elsewhere. A.1 Ionisation Chambers An ionisation chamber is a detector with a gas-filled region, which measures the amount of ionisation produced by a charged particle passing through the gas. Neutral © Springer International Publishing Switzerland 2016 161 C. Grupen and M. Rodgers, Radioactivity and Radiation, DOI 10.1007/978-3-319-42330-2 162 Appendix A: How Can We Detect Radiation? Fig. A.1 Sketch of an ionisation chamber particles can also be detected by this device via secondary charged particles (those that result from the interaction of the initial neutral ones with electrons or nuclei). The charged particles move through the gas, and strike gas molecules, liberating electrons and leaving ions: i.e. they produce more charged particles. The number of charged particles can be measured using an electric field, which attracts the oppositely charged particles in opposite directions onto conducting plates (the electrodes), from which the signals caused by the particles can be recorded. In the simplest case, an ionisation chamber consists of a pair of parallel electrodes mounted in a gas-tight container that is filled with a gas mixture (see Figure A.1). A charged particle produces electron-ion pairs along its track, and the electrons drift through the gas towards the positive electrode, while the ions drift towards the negative electrode. The number of charge carriers created depends on the particle type and energy, and on the gas used. The parallel electrodes of the ionisation chamber are initially charged to a certain voltage, producing a homogeneous electric field, i.e. one which is the same strength and points in the same direction at all positions within the field. The drifting charge carriers induce an electric charge on the electrodes, which leads to certain change of the voltage. This voltage change has contributions from the much lighter, fast-moving electrons and from the much heavier, slowly drifting ions. There is a significant dis- advantage, which is that the output signal depends on where the particles enter the ionisation chamber. This can be solved by introducing a Frisch grid (a third electrode, constructed to reduce the dependence), or by only permitting the particles to enter the chamber from a well-defined region. With either remedy in place, the amount of energy deposited by the particle can be deduced from the signal: and if the incoming particle is totally absorbed in the gas, the detector measures its total energy. Ionisation chambers are mainly used to detect particles with high charges, because in this case the energies deposited tend to be larger than those of singly charged Appendix A: How Can We Detect Radiation? 163 “The detector is that small because it has to detect tiny particles” particles. α particles have a charge of two units, and if coming from a radioactive source, they will typically deposit 5MeV in a 4cm layer of argon, which is easily detected. A singly charged particle, such as a β particle, passing through the same 4cm layer of argon will deposit only about 11keV,which provides about 400 electron- ion pairs. To detect such a small signal is a very difficult task! Figure A.2 shows a parallel-plate ionisation detector. The charged plates, which are on the top and bottom of the large square area, are sufficiently thin that they are transparent. The controls (for setup, reset and test mode) are next to the built-in display. The device can also be connected to a computer, so that readings can be logged. As well as parallel-plate ionisation counters, cylindrical ionisation counters are also in use. These have the positive electrode (anode) as a thin wire along the centre of the cylinder, and the shell of the cylinder as the negative electrode (cathode). Because of this cylindrical arrangement, the electric field in this case is no longer homogeneous, but is stronger closer to the anode wire and weaker towards the edges of the cylinder. The important point with ionisation chambers is that no multiplication of charge carriers occurs in the gas. Only the electrons and ions originally produced by the incoming particle are collected. 164 Appendix A: How Can We Detect Radiation? Fig. A.2 A parallel-plate ionisation chamber. (Model: DIAMENTOR SET CX, from PTW, Freiburg, Germany) A.2 Proportional Counters Proportional counters are in principle similar to ionisation chambers, but they use a higher voltage between the electrodes, giving a stronger signal due to an amplification effect. When a particle enters the container, it will ionise atoms and produce pairs of positive ions and (negative) electrons. These particles are accelerated strongly in the powerful electric field – sufficiently strongly that they themselves produce further electron-ion pairs when they strike other gas molecules. This process can continue with future generations of charged particles. These cascades of particles can eventually be measured when they strike the electrodes. Crucially, as long as ionisation remains the only significant process, the signal measured is proportional to the original amount of ionisation, but multiplied by, typically, 104 or 105. Proportional counters have a natural upper limit to their operation: at yet higher voltages, the accelerated electrons will not only ionise gas molecules, but will some- times excite them (put molecular electrons into higher energy states, without ejecting them from the molecule). De-excitation usually involves the emission of photons, which can interact with the electrodes (by the photoelectric effect), producing further charged particles and amplifying the cascade. The addition of this effect means that the counters are no longer proportional for very high voltages, and so they are not used with voltages over that level (which depends on the device, but is usually about 1000 V). Since the output signal in a proportional counter is proportional to the energy loss of the particle (or to the total energy of the particle, if the particle stops in the counter), these radiation detectors can be used for spectroscopy, i.e. to determine the distribution of energies for a group of many particles (e.g. of X rays or α particles). A multi-wire proportional chamber (MWPC) is a more advanced device, operating much like a proportional counter, but with multiple charged wires across a planar chamber. In additon to signals proportional to the energy, MWPCs provide accurate track information if the wires are stretched with relatively small separations. Their Appendix A: How Can We Detect Radiation? 165 major use as regards radiation protection is as contamination monitors: measuring the extent of contaminations over large areas. A.3 Geiger-Müller Counters For many circumstances, a strong detector response, even to low levels of signal, is important, even if the cost is that the energy deposited by the particles cannot be measured. In a Geiger-Müller counter (also called a Geiger counter or a GM counter), a still stronger electric field is used, so that the output is no longer proportional to the energy left in the chamber by the passing particle. Like some ionisation chambers, the shape of a GM counter is a cylinder, with the wall negatively charged and a central wire positively charged. Each ionising particle passing through the chamber causes a single brief discharge, and the count of these discharges gives an indication of the radiation level. In order to turn that indication into a measurement of dose, a different calibration is required for each radiation type and energy. Increasing the field strength in a proportional counter, beyond the limit where it ceases to be proportional, leads to copious production of photons during the forma- tion of the particle cascade. These photons, often in the ultraviolet range, may knock electrons out of the electrodes if they strike them (the photoelectric effect). The pho- ton may have travelled some distance (typically several millimetres) before striking the electrodes, and the electrons liberated can cause further cascades, distant from the original interaction point. Another effect with a similar consequence is the strong acceleration of the gas ions: at these energies, they can strike the walls of the cylinder (negative electrode) hard enough to cause ionisation there, also far from the initial interaction point.
Details
-
File Typepdf
-
Upload Time-
-
Content LanguagesEnglish
-
Upload UserAnonymous/Not logged-in
-
File Pages86 Page
-
File Size-