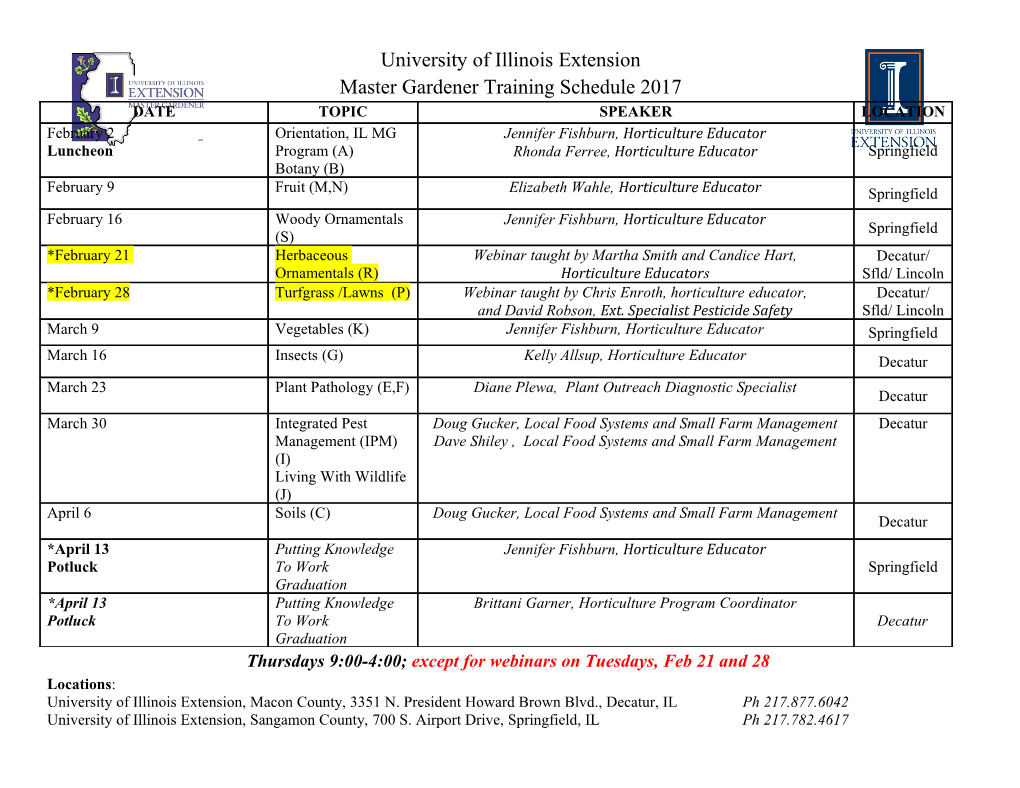
Energies 2013, 6, 1590-1603; doi:10.3390/en6031590 OPEN ACCESS energies ISSN 1996-1073 www.mdpi.com/journal/energies Article Understanding the Mechanism of Cypress Liquefaction in Hot-Compressed Water through Characterization of Solid Residues Hua-Min Liu 1,2, Ming-Fei Li 3, Sheng Yang 3 and Run-Cang Sun 2,3,* 1 School of Grain and Food, Henan University of Technology, Zhengzhou 450001, China; E-Mail: [email protected] 2 State Key Laboratory of Pulp and Paper Engineering, South China University of Technology, Guangzhou 510640, China 3 Institute of Biomass Chemistry and Technology, Beijing Forestry University, Beijing 100083, China; E-Mails: [email protected] (M.-F.L.); E-Mail: [email protected] (S.Y.) * Author to whom correspondence should be addressed; E-Mail: [email protected]; Tel./Fax: +86-10-6233-6903. Received: 26 November 2012; in revised form: 4 February 2013 / Accepted: 28 February 2013 / Published: 11 March 2013 Abstract: The mechanism of hydrothermal liquefaction of cypress was investigated by examining the effects of temperature and retention time on the characteristics of the solid residues remaining after liquefaction. The solid residues were divided into acid-soluble and acid-insoluble residues. Results showed the polymerization reactions also mainly occurred at low temperatures. The reactive fragments transformed into acid-insoluble solid residue in the form of carbon and oxygen through polymerization reactions. The process of cellulose degradation consists of two steps: an initial hydrolysis of the more solvent- accessible amorphous region and a later hydrolytic attack on the crystalline portion. Hemicelluloses were decomposed into small compounds during the initial stage of the cypress liquefaction process, and then these compounds may rearrange through polymerization to form acid-insoluble solid residues above 240 °C. The higher heating value of the solid residues obtained from liquefaction at 260–300 °C was 23.4–26.3 MJ/kg, indicating that they were suitable for combustion as a solid fuel. Keywords: liquefaction; acid-insoluble solid residue; reaction mechanisms; sugar analysis Energies 2013, 6 1591 1. Introduction Thermo-chemical conversion technologies can efficiently convert biomass to gaseous, liquid, and solid products under thermal conditions [1]. Pyrolysis and liquefaction are the typical thermo-chemical processes for the conversion of biomass to produce liquid products in high yields. During the pyrolysis process, biomass is heated in the absence of air, forming bio-oils and gaseous products [2]. Liquefaction technology as a widely used method has a lower process temperatures as compared to pyrolytic conversion. In liquefaction processes, biomass depolymerizes in the presence of organic reagents to form useful chemicals [3,4]. The bio-oils cannot be used as transportation fuels directly without further treatment due to their high oxygen and water contents. Catalytic pyrolysis liquefaction is a promising way to improve bio-oil quality by removing oxygen, while also increasing the calorific value and stability [5]. However, the use of environmentally friendly and low energy-intensive approaches is highly desired. Hot-compressed and super/subcritical water have been widely studied and developed as technologies for the conversion of biomass to clean fuel and valuable chemicals [6,7]. The main advantage of these technologies is that drying process is not required for the conversion of wet biomass, because the water contained in biomass served as solvent as well as reactant [7]. Detailed data for bio-oils are good for understanding the mechanism of biomass. In recent years, although bio-oils have been analyzed by GC-MS, FT-IR, and NMR in many reports [5,6,8], the degradation of biomass cannot be easily described by detailed chemical reaction pathways with well-defined single reaction steps. This is mainly due to the complexity of the bio-oil components, which include acids, alcohols, aldehydes, ketones, esters, heterocyclic derivatives, and phenolic compounds as products of the degradation of biomass [9]. Another reason for the difficulty in describing the chemical reaction pathways is that the liquefaction is mainly a heterogeneous process, which proceeds inside and on the surface of biomass particles [10]. Numerous works use thermo-gravimetric analysis to determine thermal decomposition of biomass mechanisms and estimate the amounts of biogas (such as methane and hydrogen) produced during the pyrolsis process [11,12]. However, the analytical technology cannot investigate the effect of solvent on the thermal characteristics and mechanism of biomass liquefaction. Although the liquefaction of biomass using different solvents has been investigated, there is little information on the thermal behavior of solvents in these liquefaction processes. Many researchers have also evaluated the characteristics of the solid residue from hydrothermal liquefaction of biomasses by using FT-IR [13–15]. However, the biomass is a polymer with a particularly complex structure, and therefore, FT-IR alone is not enough to study the hydrothermal liquefaction of biomass. However, more information from the infrared analysis of solid residues may help to better understand the liquefaction processes. To the best of our knowledge, in the previous investigations focused on the evaluation of the characteristics of whole solid residues by using techniques such as FT-IR, X-ray diffraction, and NMR few attempts have been made to first divide the solid residues into different fractions, and then further investigate the characteristics of these various fractions. In the present study, the solid residues obtained from hydrothermal liquefaction of cypress under different conditions (temperature and retention time) were divided into acid-soluble and acid-insoluble solid residues by hydrolysis with dilute sulfuric acid, and then the acid-insoluble solid residues further Energies 2013, 6 1592 divided into carbon, hydrogen, and oxygen by the elemental analyzer. The effects of temperature and retention time on the yields of different fractions were studied. Additionally, the solid residues (included acid-soluble and acid-insoluble solid residues) were characterized by FT-IR, X-ray diffraction, sugar analysis, and elemental analysis to help understand the mechanisms of the hydrothermal liquefaction process. 2. Experimental Section 2.1. Materials Cypress was collected from Henan Province in China. The feedstock was milled to obtain fine powder by passing through 40-mesh. The powder was dried at 105 °C for 24 h before used. The dried cypress contained 43.2% cellulose, 26.3% hemicelluloses, 28.2% lignin, 48.9% carbon, 44.8% oxygen, 6.0% hydrogen, 0.3% nitrogen, and 2.5% ash (on a dry weight basis). The chemicals used were analytical reagent grade. 2.2. Apparatus and Experimental Procedure Cypress sample (10 g) and de-ionized water (100 mL) were placed in a 1 L stainless steel autoclave equipped with a magnetic stirrer (Parr Instrument Company, Moline, IL, USA). The details of the liquefaction experiments were described previously [16]. The scheme of the product separation and analysis approach is shown in Figure 1. The reaction mixture was filtered to separate the water-soluble fraction and then the water-insoluble fraction was extracted with acetone in an extraction apparatus until the solvent in the thimble became colorless. The acetone-insoluble fraction was dried at 105 °C and then weighed, and this was defined as solid residue (SR). The carbohydrates present in the SRs obtained at different conditions were removed by hydrolysis with 3% H2SO4 for 4 h at 100 °C. Therefore, the solid residues were further divided into acid-soluble solid residue (ASSR) and acid-insoluble solid residue (AISR). The acid-insoluble solid residues were further divided into carbon, oxygen, and hydrogen by the elemental analyzer. The yield of solid residue was calculated on a dry-ash-free basis and each experiment was repeated twice. 2.3. Analysis The carbon, hydrogen, and nitrogen contents of cypress and solid residues were analyzed using an Elemental Analyzer (Elementar Analysen Syetem GmbH, Hanau, Germany). The oxygen content was estimated based on the assumption that the samples only contained the elements carbon, hydrogen, nitrogen, and oxygen. The higher heating values (HHV) of the samples were calculated based on Dulong formula, as described previously [16]. The SRs were evaluated using an X-ray diffractometer to determine the degree of crystallinity. X-ray diffractometry in reflection mode was conducted using a X-ray diffractometer (Shimadzu XRD-6000, Tokyo, Japan) with Cu Ká radiation source (ë = 0.154 nm) at 40 kV and 30 mA. The crystallinity index (CrI) was calculated based on the formula as reported in a previous paper [17]. The neutral sugar composition of the cypress and SRs obtained from hydrothermal liquefaction was determined by hydrolysis with 3% H2SO4 for 2.5 h at 105 °C. The liberated neutral sugars were Energies 2013, 6 1593 analyzed by high performance anion exchange chromatography (Dionex ICS-3000, Sunnyvale, CA, USA) and a Carbopac PA-1 ion exchange column (4 × 250 mm). Fourier transform infrared spectrometer (FT-IR) analysis of raw cypress, acid-insoluble lignin, SRs, and AISRs were carried out on a Nicolet iN10 FT-IR spectrophotometer (Madison, WI, USA) equipped with a liquid nitrogen-cooled MCT detector. The raw cypress material was heated at 650 °C for 6 h to determine the ash content. Figure 1. A brief scheme of the product separation and analysis approach. Cypress Liquefaction Washing with water X‐ray diffraction and acetone Solid residue FT‐IR Elemental analysis Hydrolysis Acid‐soluble Elemental Acid‐insoluble FT‐IR solid residue analysis solid residue Sugar analysis Carbon Hydrogen Oxygen 3. Results and Discussion 3.1. The Yield of Solid Residue 3.1.1. Effect of Temperature on Yield of Solid Residue The yields of the SR obtained from hydrothermal liquefaction of cypress at various temperatures are shown in Figure 2a. The lower the temperature and pressure, the higher the SR yields. The SR yield firstly decreased from 85.9 to 36.2% as the final temperature increased from 180 to 260 °C.
Details
-
File Typepdf
-
Upload Time-
-
Content LanguagesEnglish
-
Upload UserAnonymous/Not logged-in
-
File Pages14 Page
-
File Size-