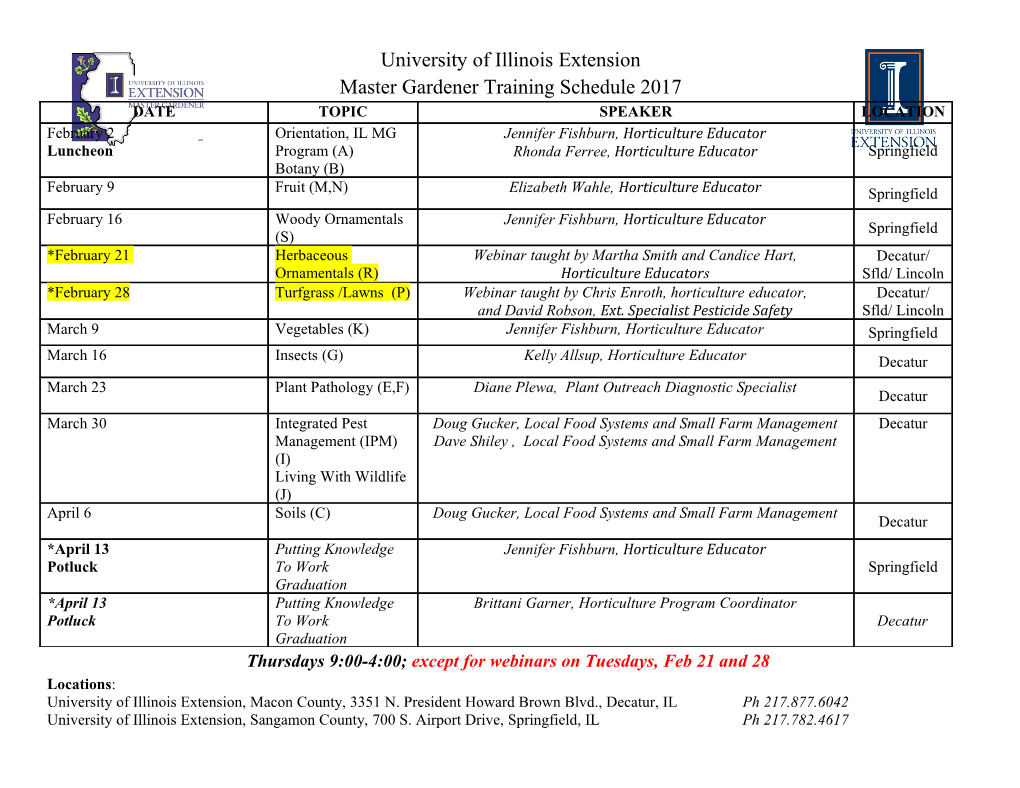
Antimalarials inhibit hematin crystallization by unique SEE COMMENTARY drug–surface site interactions Katy N. Olafsona, Tam Q. Nguyena, Jeffrey D. Rimera,b,1, and Peter G. Vekilova,b,1 aDepartment of Chemical and Biomolecular Engineering, University of Houston, Houston, TX 77204-4004; and bDepartment of Chemistry, University of Houston, Houston, TX 77204-5003 Edited by Patricia M. Dove, Virginia Tech, Blacksburg, VA, and approved May 9, 2017 (received for review January 3, 2017) In malaria pathophysiology, divergent hypotheses on the inhibition assisted by a protein complex that catalyzes hematin dimerization of hematin crystallization posit that drugs act either by the (45). In a previous paper, we reconciled these seemingly opposite sequestration of soluble hematin or their interaction with crystal viewpoints by suggesting that β-hematin crystals grow from a thin surfaces. We use physiologically relevant, time-resolved in situ shroud of lipid that coats their surface (46), a mechanism that surface observations and show that quinoline antimalarials inhibit is qualitatively consistent with experimental observations (47). β-hematin crystal surfaces by three distinct modes of action: step Driven by the evidence favoring neutral lipids as a preferred en- pinning, kink blocking, and step bunch induction. Detailed experi- vironment for hematin crystallization in vivo (46), we use a sol- mental evidence of kink blocking validates classical theory and dem- vent comprising octanol saturated with citric buffer (CBSO) at onstrates that this mechanism is not the most effective inhibition pH 4.8 as a growth medium. pathway. Quinolines also form various complexes with soluble he- Recent observations of hematin crystallization from a bio- matin, but complexation is insufficient to suppress heme detoxifica- mimetic organic medium demonstrated that it strictly follows a tion and is a poor indicator of drug specificity. Collectively, our classical mechanism of growth (48), where new layers are gener- findings reveal the significance of drug–crystal interactions and ated by 2D nucleation and advance by the attachment of solute open avenues for rationally designing antimalarial compounds. molecules. This finding allowed identification of the rate of nu- cleation of new layers, J2D, and the velocity of advancing steps v as malaria | P. falciparum | crystallization inhibition | hematin crystals | heme the main quantitative measures of β-hematin crystal growth and detoxification suggested criteria to differentiate the specificity and efficacy of growth inhibitors that bind to β-hematin crystal surfaces (48). The any pathological conditions are understood within the physiological relevance of these conclusions is reinforced by the Mrealms of molecular biology and biochemistry. An impor- observation that the morphology of the grown crystals is identical tant class of diseases, whose pathology involves the formation of to biological hemozoin (28), and their growth rate is similar to solid or liquid condensate, stands to benefit from the introduction those observed in parasites (42, 48). of concepts and mechanisms from physics and materials science To establish whether drug–crystal interactions are the dominant (1–3). A prominent example is the formation of crystalline mechanism of hematin growth inhibition, we monitor and quantify hemozoin, a vital component of malaria parasite physiology (4–7). the processes of hematin crystallization by time-resolved in situ Malaria infection starts with a mosquito bite introducing Plas- atomic force microscopy (AFM). We use CBSO as the solvent (48). modium sporozoites, which invade the host’s liver cells (Fig. 1). To this growth solution, we introduce six common antimalarial Within 2 wk, merozoite-stage parasites invade the erythrocytes, drugs: quinine (QN), chloroquine (CQ), pyronaridine (PY), amo- where they catabolize hemoglobin and release Fe(II) heme (5). diaquine (AQ), mefloquine (MQ), and artemisinin (ART). We find The released heme rapidly oxidizes to Fe(III) hematin, which is that in the presence of any of the tested drugs, the basal {100} toxic to the parasite in its free state (6). The main mechanism of CHEMISTRY heme detoxification (8) implemented by the parasite is seques- Significance tration as nontoxic hemozoin crystals (9–12) (Fig. 1C). Hematin crystallization (the synthetic analog of hemozoin has been referred Approximately 3.2 billion people are at risk for malaria. The re- to as β-hematin) (7) has been the most successful molecular target sistance of malaria parasites to current advanced multidrug treat- for antimalarial drugs (13, 14). Malaria parasite resistance to ments, recently recorded to spread outofSoutheastAsia,hasraised current drugs (15–18), including the most advanced line of anti- concerns of dire public health consequences. We demonstrate that malarial defense, artemisinin (19–24), has renewed the impetus to antimalarial drugs suppress heme detoxification in the malaria elucidate the pathways of parasite suppression (25–27). parasites in a manner that is counter to the prevailing hypothesis in It is generally accepted that the quinoline class of antimalarials the field. We find that quinoline-class drugs work by specific in- (quinine, chloroquine, amodiaquine, and others) work by sup- teractions with β-hematin crystals, which are the by-product of pressing hematin crystallization (8, 13, 21, 25, 26, 28, 29). The heme detoxification within the digestive vacuole of the parasites. prevailing hypothesis is that drugs decrease the activity of soluble We also identify specific drug adsorption sites on crystal surfaces. hematin by forming noncrystallizable complexes (13, 30). An These insights may potentially spur development of antimalarial alternative inhibition mechanism, involving drug–crystal in- – drugsthatovercomeparasiteresistancethrougharationalap- teraction, has been suggested by experimental evidence (29, 31 proach, far superior to currently used combinatorial methods. 38) and theoretical models (39, 40). A controversy surrounds the environment of hematin crystal- Author contributions: J.D.R. and P.G.V. designed research; K.N.O. and T.Q.N. performed lization in the parasite digestive vacuole (DV). A group of au- research; K.N.O., T.Q.N., and P.G.V. analyzed data; and J.D.R. and P.G.V. wrote the paper. thors has argued that several neutral lipids are present in the DV The authors declare no conflict of interest. as mesoscopic phases that accumulate hematin and serve as This article is a PNAS Direct Submission. – crystallization medium (41 43); this scenario has been supported See Commentary on page 7483. by numerical modeling (44). High-resolution electron micros- 1To whom correspondence may be addressed. Email: [email protected] or vekilov@ copy and X-ray tomography, however, have failed to detect lipid uh.edu. phases larger than 25 nm, interpreted in favor of growth from the This article contains supporting information online at www.pnas.org/lookup/suppl/doi:10. aqueous environment (10–12), where crystallization may be 1073/pnas.1700125114/-/DCSupplemental. www.pnas.org/cgi/doi/10.1073/pnas.1700125114 PNAS | July 18, 2017 | vol. 114 | no. 29 | 7531–7536 Downloaded by guest on September 28, 2021 developed means to reduce CQ concentration within the DV to levels that permit effective heme detoxification. Besides step pinning, which suppresses J2D and slows down v, the drug PY appears to follow an additional mechanism* of action. AFM observations reveal a decrease in v along the b crystallo- graphic direction in response to increasing PY concentration that * is more pronounced than along the c direction. Correspondingly, the islands in Fig. 2E exhibit exaggerated ellipticity, with a c/b ratio ∼ of *8, compared with 2.7 in pure solutions (51). The sensitivity of b the -oriented step growth to PY* concentration suggests that this drug preferentially attaches to b steps, blocking the growth sites available for hematin incorporation. An idealized schematic* of this interaction is presented in Fig. 2G. The hindered b steps form strips of high-density step bunches, Fig. 2E and SI Appendix, Fig. S3 (52–54), which amplifies the PY inhibition due to competitive supply of solute to closely spaced steps (51). vðcDÞ profiles similar to those in Fig. 2 B and C are compatible with an alternative mechanism of growth inhibition, whereby inhibitors incorporate in the crystals lattice. The resulting lattice strain increases the chemical potential of the crystal, which raises Fig. 1. Lifecycle of malaria parasites and hemozoin formation. (A) In human hosts, the lifecycle of malaria parasites consists of liver and red blood cell (RBC) stages. The characteristic times after RBC invasion for parasite growth and division, RBC rupture, and transfer of infection are displayed. (B) Sche- matic rendering of RBCs. (C) Parasite inhabiting an RBC with a nucleus (N) and a DV containing hemozoin crystals. (D) SEM image of a hematin crystal grown in vitro. (Scale bar, 5 μm.) β-hematin faces grow via a classical layer mechanism, analogous to the one observed in pure CBSO solutions (48). Results and Discussion Molecular Mechanisms of Drug Action. Three of the tested drugs, QN, CQ, and PY, depicted in Fig. 2A, cause a sharp decay of J2D (Fig. 2B) accompanied by a monotonic decrease in v relative to its value v0 in solutions of pure hematin with concentration cH = 0.28 mM, at supersaturation σ = lnðcH =ceÞ ≅ 0.56, where ce = 0.16 mM is the solubility at 28 °C, the temperature in the AFM liquid cell (48)
Details
-
File Typepdf
-
Upload Time-
-
Content LanguagesEnglish
-
Upload UserAnonymous/Not logged-in
-
File Pages6 Page
-
File Size-