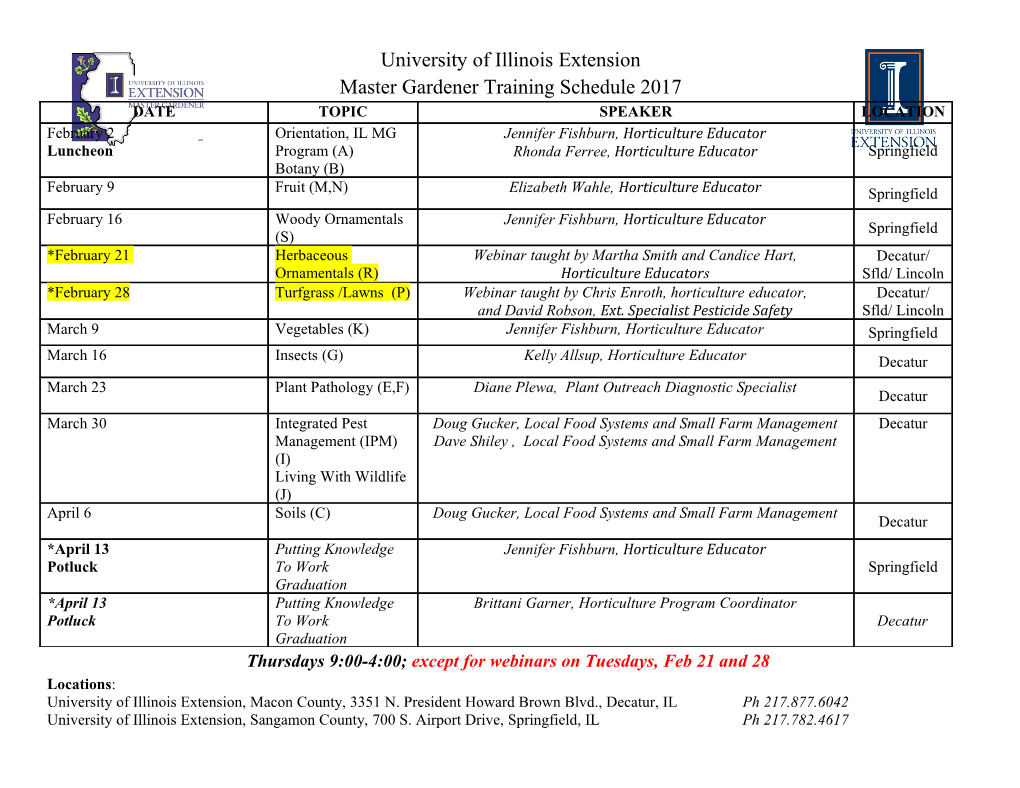
Electrochimica Acta 56 (2011) 5142–5150 Contents lists available at ScienceDirect Electrochimica Acta journal homepage: www.elsevier.com/locate/electacta Electrodeposition of cobalt–yttrium hydroxide/oxide nanocomposite films from particle-free aqueous baths containing chloride salts K.M. Sivaraman a, O. Ergeneman a, S. Pané a,∗, E. Pellicer b,∗∗, J. Sort c, K. Shou a, S. Surinach˜ b, M.D. Baró b, B.J. Nelson a a Institute of Robotics and Intelligent Systems (IRIS), ETH Zürich, CH-8092 Zürich, Switzerland b Departament de Física, Facultat de Ciències, Universitat Autònoma de Barcelona, E-08193 Bellaterra, Spain c Institució Catalana de Recerca i Estudis Avanc¸ ats (ICREA) and Departament de Física, Universitat Autònoma de Barcelona, E-08193 Bellaterra, Spain article info abstract Article history: The feasibility of growing nanostructured films composed of cobalt and yttrium hydroxide/oxide phases Received 11 December 2010 by electrodeposition is demonstrated. Particle-free aqueous solutions containing YCl3 and CoCl2 salts and Received in revised form 13 March 2011 glycine were used. The incorporation of yttrium compounds into the cobalt deposit was achieved using Accepted 14 March 2011 pulse deposition (t = 0.1 ms, t = 0.9 ms) and for cathodic pulses higher than −500 mA cm−2. Deposits Available online 22 March 2011 on off obtained were crack-free, typically with 1–5 wt% yttrium, and exhibited morphologies markedly differ- ent from the ones shown by pure cobalt deposits. Moreover, yttrium-rich films (up to 30 wt% Y) could be Keywords: deposited under certain conditions, though incipient cracking developed in this case. X-ray photoelec- Nanocomposite Cobalt tron spectroscopy analyses revealed that Y(OH)3/Y2O3 compounds were present in the films. From the Yttrium hydroxide/oxide structural viewpoint, the composites exhibited a partially amorphous/nanocrystalline character, with Pulse deposition the crystalline fractions originating from the hexagonal-close packed structure of ␣-Co. A refinement of X-ray photoelectron spectroscopy the ␣-Co crystallite size was observed in deposits containing higher weight percentage of yttrium com- pounds. Nanoindentation tests revealed that hardness increased with the yttrium content. This result can be explained by taking into account both the presence of intrinsically hard oxide phases and the effects promoted by incorporation of yttrium hydroxides/oxides on the ␣-Co matrix (namely, grain-refining and higher concentration of stacking faults). © 2011 Elsevier Ltd. All rights reserved. 1. Introduction surrounding the particles [6]. As a consequence, the anticipated advantageous chemical and/or physical properties of the com- Nanocomposite coatings consisting of ultra-fine ceramic parti- posite coatings are often not obtained. Furthermore, hydration cles (e.g., Al2O3, SiO2, SiC, TiN, AlN) embedded in a metal matrix forces can also hinder particle co-deposition [7]. Suitable surfac- have become the focus of widespread research in recent years tants can improve the stability of the suspension by increasing the due to their superior properties compared to purely metallic films. wettability of suspended particles, while enhancing the electro- Benefits include high specific heat, optical non-linearity, novel static adsorption of the dispersed particles on the cathode surface magnetic properties, enhanced mechanical behavior (large hard- by increasing their positive charge [8]. Nevertheless, there are ness and wear resistance) and good corrosion resistance, amongst also drawbacks associated with the use of surface-active agents. others [1–5]. Surfactants can get adsorbed on the cathode surface leading to Nanocomposite coatings can be produced by various meth- unfavorable changes in the mechanical properties of the electrode- ods, including electrodeposition. Electrodeposited nanocomposite posit, such as high internal residual stress or brittleness [9]. Due coatings are generally obtained by suspending charged ceramic to these reasons, the search for new approaches for synthesizing nanoparticles in the electrolyte and co-depositing them with the heterogeneous, multi-phase deposits with enhanced and tunable metal [1–5]. However, this method suffers from some drawbacks. properties is highly desirable. Nanoparticles can easily agglomerate due to the compressive effect Cathodic electrodeposition of certain ceramic materials from caused by the high ionic strength on the diffuse double layer their metal salts, including metal oxides/hydroxides or complex oxide compounds, has been demonstrated over the last few years [10–14]. In particular, deposition of yttrium hydroxide has received ∗ particular attention because it enhances the corrosion resistance of Corresponding author. Tel.: +41 44 632 33 12. ∗∗ Corresponding author. Tel.: +34 93 581 14 01; fax: +34 93 581 21 55. several metals and alloys such as carbon steel, stainless steel, zinc, E-mail addresses: [email protected] (S. Pané), [email protected] (E. Pellicer). bronze, aluminium and magnesium alloys [15]. Research on Y(OH)3 0013-4686/$ – see front matter © 2011 Elsevier Ltd. All rights reserved. doi:10.1016/j.electacta.2011.03.058 K.M. Sivaraman et al. / Electrochimica Acta 56 (2011) 5142–5150 5143 deposition has been primarily motivated by the need to replace Table 1 −3 Cr(VI) based corrosion inhibitors by eco-friendly protective layers, Detailed composition of the employed baths, in mol dm . given that Cr(VI) is extremely toxic and carcinogenic [16]. In addi- Bath YCl3·6H2O CoCl2·6H2O Glycine (C2H5NO2) KCl H3BO3 tion to the corrosion inhibiting performance, electrodeposition of 1 0 0.1 Y(OH)3 has also been a topic of research because it can be con- 2 1 0.05 0.15 1 0.16 verted into Y2O3 (yttria) after an appropriate thermal treatment. 3 1 0.1 Yttria is highly resistant to most chemicals and has a melting point 4 1.5 0.1 of approximately 2400 ◦C. It also exhibits a low vapor pressure, opti- cal transparency in the infrared region, high corrosion and thermal resistance, and high strength and fracture toughness [17–20]. This wherein the [Co(II)] was much smaller than the [Y(III)] (Table 1). KCl was used as a supporting electrolyte and boric acid as a buffering has prompted the use of yttria in a variety of industrial applica- ◦ tions, including optical sensors, cutting tools, protective coatings, agent. The working temperature was 60 C. This temperature was and solid oxide fuel cell (SOFC) interconnects [20–23]. selected on the basis that yttrium chemically behaves as a RE ele- ment. RE metal deposition is generally more favorable above room Cathodic electrodeposition of Y(OH)3 can be performed from either organic (typically, water–alcohol mixtures) or fully aqueous temperature [28]. The pH was kept at 4 for all the electrodeposition solvents. According to the early work by Switzer, the formation of experiments. × an electrogenerated base leads to the precipitation of Y(III) from The working electrode consisted of 5 mm 6 mm silicon chips the electrolyte as a hydroxide film on the surface of the cathode (crystal orientation 1 0 0), on top of which a titanium adhesion [24]. From ecological and health perspectives, fully aqueous elec- layer of 50 nm and a copper seed-layer of 500 nm had been suc- trolytes are certainly more desirable. However, there is a dearth cessively deposited through e-beam evaporation. Evaporation was performed using an E306A coating apparatus controlled by an e- of literature concerning the aqueous electrodeposition of Y(OH)3 Vap CVS-6 system. Before being used for electrodeposition, the films. In [25,26], Y(NO3)3 and YCl3 salts dissolved in water were copper surface was degreased by first dipping it in acetone followed used to cathodically deposit Y(OH)3 thin films and nanospheres. In ◦ by iso-propanol and then stripped of oxide and organic residues both works, Y(OH)3 was thermally treated at 600 C in air to obtain crystalline yttria. by dipping in 10% H2SO4 solution. The three electrodes were con- To date, attempts to incorporate yttrium oxides/hydroxides nected to a Gamry Reference 3000 potentiostat/galvanostat which into metallic electrodeposits have proven difficult. The production was controlled by the Gamry Framework software during gal- of nickel–yttria composite coatings by direct current electroplat- vanostatic and linear sweep voltammetry (LSV) experiments and ing from a Watts nickel plating bath containing variable amounts by the Gamry VFP-600 software during pulsed electrodeposition. −2 of micron-sized yttria particles suspended in the electrolyte was The charge density was kept at 20 C cm across all depositions. explored by McCormack et al. [27]. However, there was little or no Deposits were obtained by galvanostatic pulse plating with a − − −2 yttria inclusion in the deposits for the investigated range of yttria cathodic pulse (CP) (from 50 to 1500 mA cm ) with a duration loadings (1–5 g dm−3). The authors reported only on the effects of ton = 0.1 ms followed by an open circuit potential (OCP) with a caused by the presence of yttria dispersed in the electrolytic bath duration of toff = 0.9 ms (Fig. 1). The data acquisition frequency was on the Ni deposit texture and morphology. Hence, incorporation 10 kHz which provided 10 data points for each cycle. The deposition of a useful amount of yttrium-based compounds into electroplated was carried out under quiescent conditions. metallic films remains a challenge and requires investigation. The morphology of the deposits was observed using Zeiss NVi- The aim of the present work is to demonstrate the feasibility sion 40 and Zeiss Merlin scanning electron microscopes (SEM). of producing yttrium-containing cobalt films by electrodeposi- The chemical composition of the deposits was analyzed by energy tion from aqueous solutions. The novelty of the present synthetic dispersive X-ray spectroscopy (EDX). Data reported are based on approach relies on incorporating yttrium oxides/hydroxides into the averaging of four measurements on three replicas per sam- nanocrystalline Co films from a fully aqueous electrolyte contain- ple. The yttrium content is calculated by taking into account the weight percentages of cobalt and yttrium elements (without con- ing both YCl3 and CoCl2 salts.
Details
-
File Typepdf
-
Upload Time-
-
Content LanguagesEnglish
-
Upload UserAnonymous/Not logged-in
-
File Pages9 Page
-
File Size-