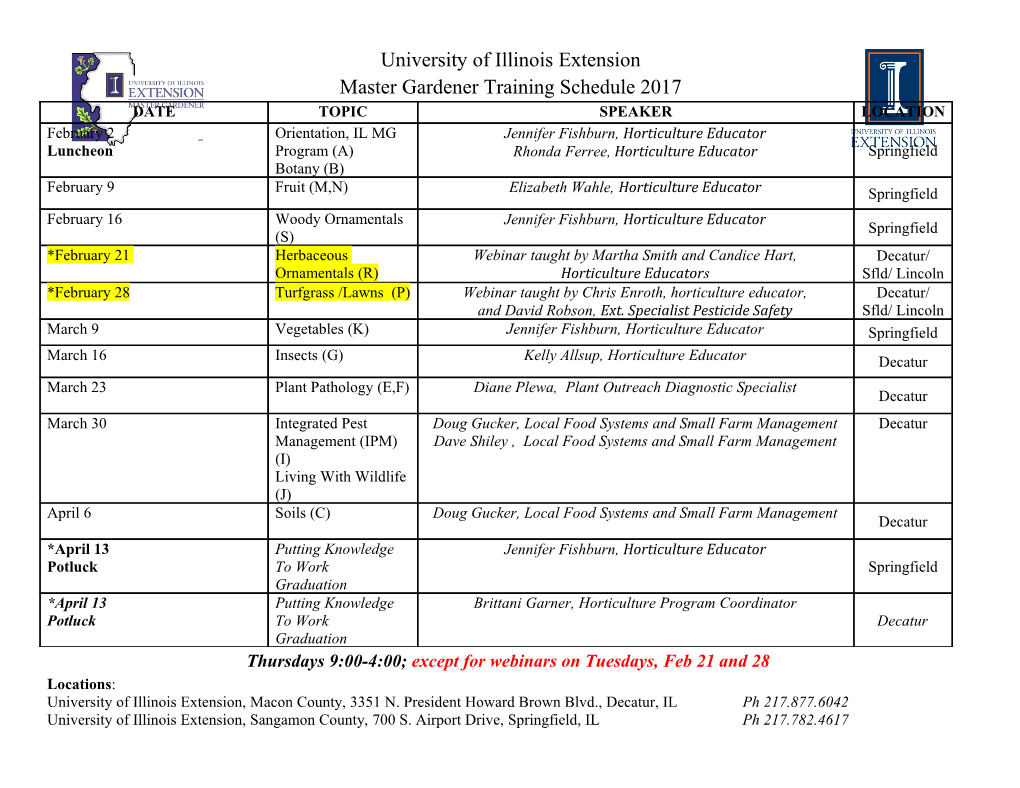
SYNTHESIS AND CHARACTERIZATION OF LOW DIMENSIONALITY CARBON NANOSTRUCTURES Dissertation Submitted to The School of Engineering of the UNIVERSITY OF DAYTON In Partial Fulfillment of the Requirements for The Degree Doctor of Philosophy in Materials Engineering by Michael Hamilton Check UNIVERSITY OF DAYTON Dayton, Ohio December, 2013 SYNTHESIS AND CHARACTERIZATION OF LOW DIMENSIONALITY CARBON NANOSTRUCTURES Name: Check, Michael Hamilton APPROVED BY: __________________________ __________________________ Andrey A. Voevodin, Ph.D. Douglas S. Dudis, Ph.D. Advisory Committee Chairman Advisory Committee Member Professor Professor Materials Engineering Department Materials Engineering Department __________________________ __________________________ Paul T. Murray, Ph.D. Scott A. Gold, Ph.D. Advisory Committee Chairman Advisory Committee Member Professor Professor Materials Engineering Department Chemical Engineering Department __________________________ __________________________ John G. Weber, Ph.D. Tony E. Saliba, Ph.D. Associate Dean Dean, School of Engineering School of Engineering & Wilke Distinguished Professor ii © Copyright by Michael Hamilton Check All rights reserved 2013 iii ABSTRACT SYNTHESIS AND CHARACTERIZATION OF LOW DIMENSIONALITY CARBON NANOSTRUCTURES Name: Check, Michael Hamilton University of Dayton Advisor: Dr. Andrey A. Voevodin Synthesizing nanostructures represents a critical technology in the field of materials science. The ability to actively control the structure and composition of matter have allowed some of the greatest scientific achievements in the last decade. This document explores the synthesis and characterization of various carbon nanostructures (e.g. DNA and doped fullerene materials). Furthermore, this document addresses how these materials can be processed into low dimensional solids while maintaining compositional integrity. Processing methods include Matrix Assisted Pulsed Laser Deposition (MAPLE), thermal evaporation, and Chemical Vapor Deposition (CVD). The synthesized bulk structures were analyzed using physical and structural measurements. Project conclusions provided insight into the unique structure-property relationships in these materials. iv Dedicated to my parents v ACKNOWLEDGEMENTS I would like to acknowledge my Ph.D. advisory committee members for guidance and assistance with preparing my dissertation: Dr. Andrey Voevodin (advisor), Dr. Douglas Dudis (advisor TE work), Dr. Terry Murray, and Dr. Scott Gold. In addition, I would like to thank the following people from AFRL/RX (Materials and Manufacturing Directorate) for technical assistance and discussion: Scott Apt, John Bultman, Steve Fairchild, Jianjun Hu, Michael Jespersen, Chris Muratore, Jose Nainaparampil, Steve Patton, Art Safriet, Adam Waite, Willem Wennekes, and Bob Wheeler. vi TABLE OF CONTENTS ABSTRACT………………………………………………………………………...........iv DEDICATION……………………………………………………………………………v ACKNOWLEDGEMENTS……………………………………………………………...vi LIST OF ILLUSTRATIONS……………………………………………………………xi LIST OF TABLES…………………………………………………………………….xviii LIST OF EQUATIONS………………………………………………………………..xix LIST OF ABBREVIATIONS AND NOTATIONS…………………………………..xxi CHAPTER I: INTRODUCTION………………………………………………………….1 CHAPTER II: BACKGROUND………………………………………………………….3 2.1 Physical Properties of Carbon Nanostructured Materials…….……………….3 2.1.1 Introduction………………….………………………………………3 2.1.2 Material Properties of Carbon Nanostructured Materials……….…..4 2.1.3 Concluding Remarks……………………………………………….22 2.2 Generalized Model for Thermal and Electrical Transport in Nanostructures..22 2.2.1 Introduction……………………………………………...…………22 2.2.2 Thermal Transport Phenomena…………………………………….23 vii 2.2.3 Electrical Transport Phenomena…………...………………………31 2.2.4 Concluding Remarks……………………………………………….38 2.3 Physical and Chemical Processing of Nanostructures……………………….38 2.3.1 Introduction………………………………………………………...38 2.3.2 Physical Vapor Deposition Methods………………………………39 2.3.3 Chemical Vapor Deposition (CVD) Methods……………………...47 2.3.4 Wet Chemical Based Methods……………………………………..52 2.3.5 Concluding Remarks……………………………………………….56 2.4 Thin Film Processes………………………………………………………….56 2.4.1 Growth Modes with Thermodynamic and Kinetic Considerations..56 2.4.2 Theory of Nucleation and Growth…………………………………58 2.4.3 Types of Growth…………………………………………………...60 2.4.4 Literature Examples of Carbon Nanostructured Growth…………..62 2.5 Characterization of Nanostructured Materials……………………………….64 2.5.1 X-Ray Photoelectron Spectroscopy (XPS)………………………...65 2.5.2 X-Ray Diffraction (XRD)………………………………………….66 2.5.3 Raman Spectroscopy……………………………………………….67 2.5.4 Electron Microscopy……………………………………………….68 CHAPTER III: RESEARCH OBJECTIVES…………………………………………….71 3.1 Investigate the Structure, Chemistry, Morphology and Formation Mechanism of Carbon Nanomaterials (DNA, and C60)…………..………….71 3.1.1 Motivation for the use of DNA-CTMA………………………...….71 3.1.2 Motivation for the use of Fullerides………………………………..72 3.1.3 Motivation for Deposition Techniques…………………………….75 3.2 Correlate the structure, chemistry, morphology of carbon nanomaterials with their physical properties………………………………………………...76 3.2.1 Thermal Properties…………………………………………………76 viii 3.2.2 Electrical Properties………………………………………………..76 3.2.3 Electron-Phonon Coupling…………………………………………76 3.3 Establish a Fundamental Relationship Between the Transport Mechanism and the Structure in DNA, and C60 Based Materials………….……….…….77 CHAPTER IV: EXPERIMENTAL METHODS…………………………...……………78 4.1 Nanostructure Deposition/Formations Methods……………………………..78 4.1.1 Formation of the DNA-CTMA Complex………………………….78 4.1.2 MAPLE of DNA…………………………………………………...80 4.1.3 Thermal Evaporation of C60……………………….….……………81 4.1.4 CVD of C60 and Zinc………………………………………………82 4.2 Thermal Properties…………………………………………………………...84 4.2.1 Thermal Conductivity……………………………………………...84 4.2.2 Heat Capacity by Means of Differential Scanning Calorimetry (DSC)………………………………………………………………90 4.3 Electrical Properties………………………………………………………….91 4.3.1 Four Point Probe…………………………………………………...91 4.3.2 PPMS………………………………………………………………93 4.3.3 Potential Seebeck Microprobe (PSM)……………………………..93 CHAPTER V: RESULTS AND DISCUSSION………………………………………...96 5.1 Process Development and Nanostructure Formation Mechanisms for DNA and C60……………………………………………………………..………...96 5.1.1 MAPLE process development……………………………………..96 5.1.2 Inducing Marangoni Flow for the Reduction of Coffee Ring Evaporation………………………………...……………………..109 5.1.3 Growth of Fulleride Thin Films…………………………………..115 5.1.4 Growth of Fulleride Nanowires…………………………………..127 ix 5.2 Thermal Characterization of DNA and C60 Compounds…………………...134 5.2.1 Thermal Characterization of DNA-CTMA Films………………...134 5.2.2 Thermal Characterization of Zinc C60 Thin Films…………….….136 5.2.3 Thermal Characterization of the Zinc C60 Nanowires………...….138 5.3 Electrical Characterization of Fullerides…………………………………...139 5.3.1 Electrical conductivity of ZnxC60 Thin Films and ZnxC60 Nanowires………………………………………………………...139 5.3.2 Seebeck Coefficient of ZnxC60 Thin Films and ZnxC60 Nanowires………………………………………………………...141 5.3.3 Electron-Phonon Coupling in ZnxC60 Nanowires…………….….144 CHAPTER VI: CONCLUSIONS………………………………………………………150 6.1 Future Work………………………………………………………………...152 BIBLIOGRAPHY………………………………………………………………….......154 APPENDIX…………………………………………………………………………….172 x LIST OF ILLUSTRATIONS 1.Figure 2.1.1:Relative space filling models displaying the different types of nanostructures from zero dimensional to three dimensional.4 ............................................ 4 2. Figure 2.1.2: A) Hall – Petch plot for different nanocrystalline materials of various sizes.5 B) Schematic of Grain size dependence vs. various mechanism for deformation behavior.7 ............................................................................................................................ 6 3. Figure 2.1.3: Hardness of a nanocrystalline diamond film vs. grain size.9 ..................... 7 4. Figure 2.1.4: Elastic modulus vs. the amount of disorder in a carbon nanotube.16 ........ 9 5. Figure 2.1.5: A) the solution of a potential well in a atom B) the time dependent Schrodinger equation C) The variation in potential wells for multiple atoms.18 ............. 11 6. Figure 2.1.6: A) Potential well with the addition of multiple atoms with various atomic spacing B) Schematic displaying the collective picture of atomic band structure.18 ......................................................................................................................... 12 7. Figure 2.1.7: A) Illustration representing the density of states for various 0D, 1D, 2D, and 3D structures.19 B) This equation describes the relative density per energy state for a 3D system. C) The density per energy state is simply the derivative of the number of state divided by their energy. .......................................................................... 13 8. Figure 2.1.8: A) The normalized C K-edge absorption spectra of micron to nano sized particles of diamond. B) the calculated energy values for the conduction band edge and gap widening (insert) in small crystallites of diamond.22 .................................. 14 9. Figure 2.1.9: (TOP) the theoretical DOS for a metallic nanotube and a semiconducting nanotube. (BOTTOM) the measured differential conductance vs. applied voltage on a scanning tunneling microscope.23 .................................................... 15 10. Figure 2.1.10: A) Graphene π and π* electronic bands. Linear dispersion relations close to the K (white dots) and K’ (black dots) points of the first 2D Brillouin zone are illustrated
Details
-
File Typepdf
-
Upload Time-
-
Content LanguagesEnglish
-
Upload UserAnonymous/Not logged-in
-
File Pages209 Page
-
File Size-