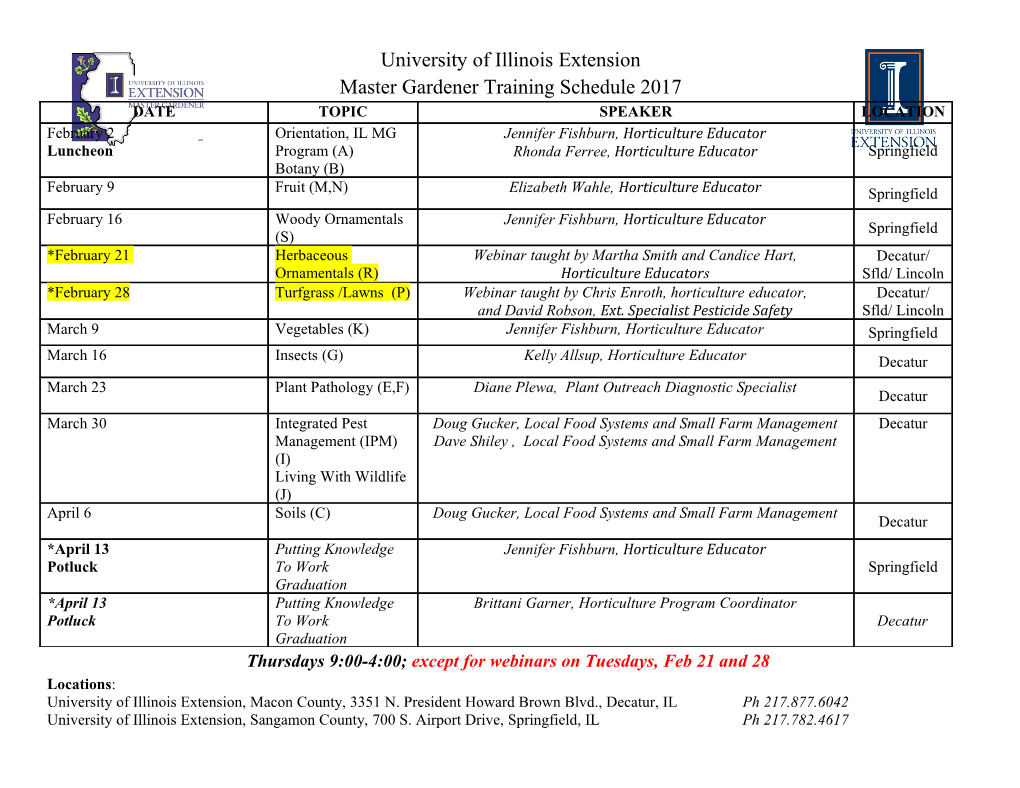
Experimental measurement of the diamond nucleation landscape reveals classical and nonclassical features Matthew A. Gebbiea,b, Hitoshi Ishiwatab,c, Patrick J. McQuadea,b, Vaclav Petrakd, Andrew Taylord, Christopher Freiwalde,f, Jeremy E. Dahlb, Robert M. K. Carlsonb, Andrey A. Foking,h, Peter R. Schreinerg, Zhi-Xun Shenb,i, Milos Nesladeke,f, and Nicholas A. Melosha,b,1 aDepartment of Materials Science and Engineering, Stanford University, Stanford, CA 94305; bStanford Institute for Materials and Energy Sciences, SLAC National Accelerator Laboratory, Menlo Park, CA 94025; cDepartment of Electrical and Electronic Engineering, Tokyo Institute of Technology, Meguro-ku, Tokyo 152-8550, Japan; dInstitute of Physics of the Czech Academy of Sciences, CZ-18221 Prague, Czech Republic; eInstitute of Materials Research, University of Hasselt, B-3590 Diepenbeek, Belgium; fInstitute for Materials Research in Microelectronics, Interuniversity Microelectronics Centre, B-3590 Diepenbeek, Belgium; gInstitute of Organic Chemistry, Justus Liebig University, D-35392 Giessen, Germany; hDepartment of Organic Chemistry, Igor Sikorsky Kiev Polytechnic Institute, 03056 Kiev, Ukraine; and iApplied Physics, Stanford University, Stanford, CA 94305 Edited by Catherine J. Murphy, University of Illinois at Urbana–Champaign, Urbana, IL, and approved July 9, 2018 (received for review March 1, 2018) Nucleation is a core scientific concept that describes the formation of pathways, additional thermodynamic barriers, and the existence new phases and materials. While classical nucleation theory is applied of distinct subcritical and postcritical regimes with differing scaling across wide-ranging fields, nucleation energy landscapes have never in nucleation rates and probabilities are key elements of these been directly measured at the atomic level, and experiments suggest updated theories. that nucleation rates often greatly exceed the predictions of classical However, there is still a large discrepancy between the theoretical nucleation theory. Multistep nucleation via metastable states could models and experimental measurements, which show that nucle- explain unexpectedly rapid nucleation in many contexts, yet exper- ation occurs much more rapidly than predicted by classical nucle- imental energy landscapes supporting such mechanisms are scarce, ation theory (8–11). Resolving the origin of such rapid nucleation particularly at nanoscale dimensions. In this work, we measured the rates remains an area of active investigation, often explained by nucleation energy landscape of diamond during chemical vapor metastable states that lower nucleation barriers (9–15) or multistep deposition, using a series of diamondoid molecules as atomically pathways. One of the central challenges has been that these es- defined protonuclei. We find that 26-carbon atom clusters, which do sential regimes have remained inaccessible experimentally. This is not contain a single bulk atom, are postcritical nuclei and measure particularly true for industrially important gas-phase reactions, such the nucleation barrier to be more than four orders of magnitude as plasma-enhanced chemical vapor deposition (PECVD) of di- smaller than prior bulk estimations. These data support both classical amond and silicon (16–19). For example, diamond is used as a and nonclassical concepts for multistep nucleation and growth functional coating in many applications(16)andisanemerging during the gas-phase synthesis of diamond and other semiconduc- material for quantum information technologies (20) and advanced tors. More broadly, these measurements provide experimental biolabeling (21). While there is a rich history of studying diamond evidence that agrees with recent conceptual proposals of multistep growth, the extreme conditions of diamond synthesis have pre- nucleation pathways with metastable molecular precursors in di- vented the measurement of diamond nucleation energetics (17, 18). verse processes, ranging from cloud formation to protein crystalli- Nucleation energy landscapes have never been measured at zation, and nanoparticle synthesis. the atomic level due to the extreme challenges associated with observing the dynamic, few-atom clusters thought to participate nucleation | diamond | nanomaterials | thermodynamics | plasma synthesis Significance lassical nucleation theory (1–4) proposes that nucleation is a Cthermally activated process, where atomic clusters, called nu- Nucleation is the limiting step for thermodynamic phase transi- clei, stochastically evolve from metastable solution to form ther- tions. While classical models predict that nucleation should be modynamically stable phases and materials. In classical models, extremely rare, nucleation is surprisingly rapid in the gas-phase nuclei are often assumed to be microscopic clusters of the final bulk synthesis of diamond, silicon, and other industrial materials. We phase, and nucleation barriers originate in a competition between developed an approach for measuring nucleation landscapes us- unfavorable surface energies and favorable bulk free energies, ing atomically defined precursors and find that diamond critical which exhibit differing scaling with particle size. Below a certain nuclei contain no bulk atoms, which leads to a nucleation barrier size, called the critical size, the unfavorable surface energy is that is four orders of magnitude lower than prior bulk estima- dominant, biasing protonuclei toward dissolution. Random energy tions. Our findings suggest that metastable molecular precursors and concentration fluctuations allow the protonuclei to grow or play a key role in lowering nucleation barriers during materials shrink diffusively within the nucleation energy landscape, occa- synthesis and provide quantitative support for recent theoretical sionally overcoming the critical nucleation barrier, leading to rapid A proposals of multistep nucleation pathways with much lower particle growth (Fig. 1 ). barriers than the predictions of classical nucleation theory. The difference in scaling between surface and volumetric en- ergies leads to two key hallmarks of classical nucleation theory: Author contributions: Z.-X.S., M.N., and N.A.M. designed research; M.A.G., H.I., P.J.M., (i) an exponential relationship between subcritical nuclei surface V.P., A.T., and C.F. performed research; J.E.D., R.M.K.C., A.A.F., and P.R.S. contributed new areas and the probability of crossing the nucleation barrier, and reagents/analytic tools; M.A.G., M.N., and N.A.M. analyzed data; and M.A.G. and N.A.M. (ii) a critical size where relative nucleation probabilities abruptly wrote the paper. increase, indicating a boundary between the subcritical regime, The authors declare no conflict of interest. where nuclei are thermodynamically biased to dissolve, and the This article is a PNAS Direct Submission. postcritical regime, where nuclei are biased to grow. Published under the PNAS license. Recent theoretical advances (5–7) have extended classical 1To whom correspondence should be addressed. Email: [email protected]. nucleation theory, for example, by including both size and den- This article contains supporting information online at www.pnas.org/lookup/suppl/doi:10. sity as order parameters to describe nucleation pathways (6) or 1073/pnas.1803654115/-/DCSupplemental. including size-dependent interfacial free energies (7). Multistep Published online August 1, 2018. 8284–8289 | PNAS | August 14, 2018 | vol. 115 | no. 33 www.pnas.org/cgi/doi/10.1073/pnas.1803654115 Downloaded by guest on October 1, 2021 A C dissoluon* growth J) r -20 1 2 adamantane diamantane * ΔG * C H POCl C H POCl i ΔG 10 15 2 14 19 2 nucleaon Energy (10 Energy barrier 0 10 14 18 22 26 3 4 Number of carbon atoms triamantane [1(2)3]tetramantane B C H POCl C H POCl ) 18 23 2 22 27 2 26 275 2 22 250 225 18 200 14 175 Carbon atoms 10 150 5 5 Surface area (A area Surface 12345 [12(1)3]pentamantane [1(2,3)4]pentamantane C H POCl C H POCl Diamondoid order, N 26 31 2 26 31 2 Fig. 1. Diamond nucleation landscape and diamondoid protonuclei. (A) Approach for measuring nanoscale thermodynamic energy landscapes during nu- cleation. Molecular nuclei of atomically defined sizes and shapes, diamondoids (28), were used to map out the nucleation energy landscape during diamond PECVD by starting at precise locations on the thermodynamic nucleation energy landscape and measuring relative nucleation probabilities. (B) Diamondoid order, N, versus the number of carbon atoms in each diamondoid (Left) and diamondoid van der Waals surface areas (Right). N is defined as 1 for ada- mantane, and each subsequent addition of a four-carbon isobutyl substituent increases N by an integer value. (C) Molecular structures of the diamondoid protonuclei, ranging from 10 to 26 carbon atoms in size, are shown below the plot. The diamondoid order, N, is shown above each chemical name. Dia- mondoids were functionalized with POCl2 self-assembly groups (29) for covalent attachment to PECVD substrates. in nucleation processes. Recently, researchers have made strides hypothetical nucleation energy landscape in Fig. 1A. Assuming that toward visualizing nucleation in liquid–liquid and colloidal systems, all of the diamondoids are located along the same nucleation where optical imaging was used to study colloids (22, 23) and pathway, the measured density of fully formed diamond nano- proteins (12, 24), since large particle sizes permit direct observation. particles, ρ, should scale as the nucleation barrier passage proba- Emerging
Details
-
File Typepdf
-
Upload Time-
-
Content LanguagesEnglish
-
Upload UserAnonymous/Not logged-in
-
File Pages6 Page
-
File Size-