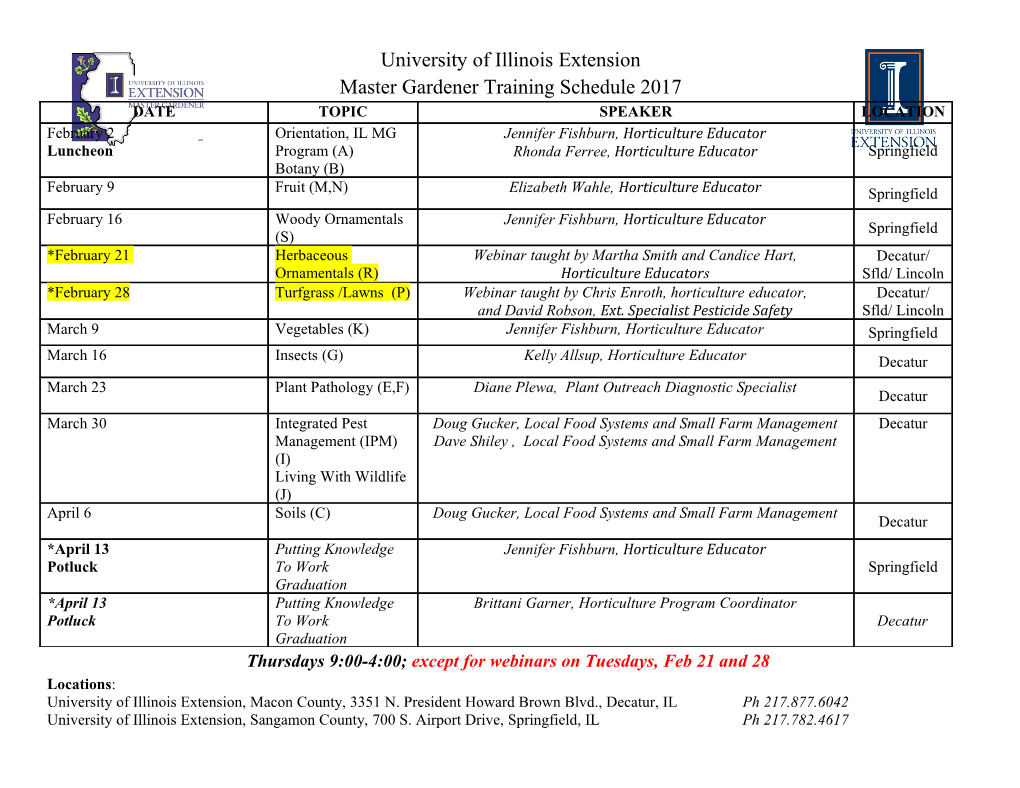
Metabolic cost as a unifying principle governing neuronal biophysics Andrea Hasenstauba,1, Stephani Ottea,b, Edward Callawaya, and Terrence J. Sejnowskia,c aCrick–Jacobs Center for Theoretical and Computational Biology, Salk Institute for Biological Studies, La Jolla, CA 92037; and bNeurosciences Graduate Program, Division of Biological Studies, and cHoward Hughes Medical Institute, Division of Biological Studies, University of California at San Diego, La Jolla, CA 92093 Edited by Eve Marder, Brandeis University, Waltham, MA, and approved May 17, 2010 (received for review December 24, 2009) The brain contains an astonishing diversity of neurons, each express- Basics of Action Potential Generation. The Hodgkin–Huxley model of ing only one set of ion channels out of the billions of potential channel action potential generation uses fast voltage-gated sodium (Na+) combinations. Simple organizing principles are required for us to channels, delayed rectifier potassium (K+) channels, and voltage- make sense of this abundance of possibilities and wealth of related independent (“leak”) potassium channels. At rest (Figs. 1A and 2A-1), data. We suggest that energy minimization subject to functional the cell’s batteries are already charged—Na+ ions are more concen- constraints may be one such unifying principle. We compared the trated outside the cell than inside (Fig. 1, red ovals), whereas K+ ions energy needed to produce action potentials singly and in trains for (Fig.1,bluediamonds)aremoreconcentratedinsidethecellthan a wide range of channel densities and kinetic parameters and ex- outside. The action potential is triggered when modest depolarization amined which combinations of parameters maximized spiking func- (Fig. 2A-2) causes the sodium channels’ activation/deactivation gates tion while minimizing energetic cost. We confirmed these results for (“m” gates, Fig. 2B, light red) to enter the “open state” (Fig. 1B). This sodium channels using a dynamic current clamp in neocortical fast increases the membrane’s permeability to Na+ ions (Fig. 1B;Fig.2C, spiking interneurons. We find further evidence supporting this hypo- red), permitting Na+ ions to passively flow down their concentration thesis in a wide range of other neurons from several species and gradient and enter the cell. This influx of positive charge (Fig. 2D,red) conclude that the ion channels in these neurons minimize energy depolarizes the membrane, bringing the membrane potential up to the expenditure in their normal range of spiking. Na+ ions’ reversal potential, ∼50 mV (Fig. 2A-3). This strong depolarization triggers the potassium channel ac- action potential | energy | optimization | ion channel | evolution tivation/deactivation gates (“n” gates, Fig. 2B, blue) to open, in- creasing the membrane’s permeability to K+ ions (Fig. 2C, blue), he mammalian genome contains genes encoding hundreds of which lets potassium exit the cell (Fig. 2D, blue). This efflux of + fl + Ttypes of ion channels, most of which can be produced in mul- K ions competes with the in ux of Na ions to control the tiple splice variants and regulated at multiple phosphorylation membrane potential; the net effect is to hyperpolarize the neuron A ’ sites by numerous intrinsic and extrinsic factors (1–4). Which (Fig. 2 -4). At the same time, the sodium channels inactivation gates (“h” gates, Fig. 1C;Fig.2B, dark red) begin to block the combination of these ion channels will be expressed by any par- ’ ticular neuron, with any particular computational or behavioral sodium channels, slowly reducing the membrane s sodium per- meability. Eventually, sufficient hyperpolarization causes the m role? Because any given electrical phenotype can be produced by and n gates both to enter the deactivated state, (Fig. 2 A-5 and many combinations of ion channels (5–8), on functional grounds B), bringing the membrane’s sodium and potassium permeabil- alone, this question is severely underconstrained. What other con- ity back to baseline levels. straints might govern ion channel expression? And can exploring These changes in gate states, channel conductances, and cur- these constraints help us understand how cells or circuits are rent flow were fueled by the energy stored in the cell’sNa+ and constructed? K+ gradients: No ATP was expended during the generation of Energy consumption may be one such constraint. The brain is the action potential itself. These gradients are now partly run one of the most energetically demanding organs in the body (9, 10); down and must be actively restored. The sodium-potassium the human brain uses more than twice as much glucose per day as pump (Na+,K+ ATPase) is primarily responsible for this resto- the heart (10). Neuronal activity—action potential generation, in- ration. It imports two K+ ions and extrudes three Na+ ions (Fig. put integration, and synaptic transmission—accounts for 50–80% 1D), at the cost of one ATP. There is thus a direct relationship of this energy use (11–14). Potential energy is stored in trans- between the number of Na+ ions that enter the cell during action membrane ion gradients, which creates a cellular battery whose potential generation and the energy cost of recovering from the maintenance accounts for most of the brain’s ATP consumption action potential. (11, 13, 15, 16). Action potential generation taps into these gra- dients and expends some of this potential energy, which needs to be Spike Cost and Spike Rate. The ionic currents underlying the action DUpper + actively restored. How can this energy be most efficiently used to potential are plotted in Fig. 2 , and the cumulative Na fl DLower generate activity or carry out computation? in ux is plotted in Fig. 2 .Notethatactionpotentialre- This question has inspired a body of research on how to enco- polarization (between times 3 and 5), not just depolarization de a signal, or perform a computation, using as few action (between times 2 and 3), accounts for a substantial portion of this potentials—and thus as little energy—as possible (17–24). Which combinations of ion channels will minimize energy cost depends NEUROSCIENCE ’ fi Author contributions: A.H. and S.O. designed research; A.H. and S.O. performed research; both on the neuron s function, such as its typical ring rate, as well A.H. contributed new reagents/analytic tools; A.H. analyzed data; and A.H., S.O., E.C., and as on the detailed kinetics of the channels (25). Here, we combine T.J.S. wrote the paper. biophysical modeling with dynamic-clamp electrophysiology to The authors declare no conflict of interest. understand the constraints on the kinetics and density of the ion This article is a PNAS Direct Submission. channels underlying action potential generation. We find that ion Freely available online through the PNAS open access option. channel expression in various species, neural structures, and cell 1To whom correspondence should be addressed. E-mail: [email protected]. types supports the hypothesis that they may be constrained to This article contains supporting information online at www.pnas.org/lookup/suppl/doi:10. minimize energy use, subject to functional requirements. 1073/pnas.0914886107/-/DCSupplemental. www.pnas.org/cgi/doi/10.1073/pnas.0914886107 PNAS | July 6, 2010 | vol. 107 | no. 27 | 12329–12334 Downloaded by guest on September 28, 2021 AB C the neuron can spike. Partial sodium channel deinactivation is required to replenish the pool of sodium channels available for action potential generation, and this deinactivation can occur only when the membrane is sufficiently hyperpolarized. Indeed, narrowing the action potential by tripling the rate of potassium channel activation (detailed in Fig. 2 E–H) does increase the rate at which the model neuron is able to spike (Fig. 3A). However, D because sodium channel inactivation accumulates slowly, sodium channels are less inactivated earlier in the action potential. If potassium channels activate earlier, sodium and potassium con- ductances will overlap more extensively (Fig. 2G). Earlier hyper- polarization will therefore be opposed by a greater sodium cur- rent flow, increasing the total Na+ influx during repolarization and thus increasing the metabolic cost of the action potential (Fig. 2H). These two factors thus imply a trade-off between the Fig. 1. (A) At rest, both sodium channels (light red) and K+ channels (light energy cost of action potential generation, particularly repolari- blue) are closed, Na+ ions (red ovals) are concentrated extracellularly, and K+ zation, and neurons’ functional capacity—their ability to spike ions (blue diamonds) are concentrated intracellularly. (B) Depolarization rapidly or their bandwidth. causes Na+ channels to open, permitting Na+ ions to flow down their con- centration gradients into the cell. This influx of positive charge depolarizes Kinetics, Rates, and Costs. A + + For the model shown in Fig. 3 ,sys- the neuron. (C) This depolarization causes K channels to open, letting K tematically speeding (curves and points on curves labeled “a” in leave the cell, hyperpolarizing the membrane potential; at the same time, + Fig. 3A) or slowing (curves and points on curves labeled “c” in Fig. depolarization causes Na channel inactivation gates (dark red ball and A chain) to close, limiting Na+ influx. Eventually the hyperpolarization is suf- 3 ) the time constants of potassium channel activation speeds or ficient to close and deinactivate the Na+ channels and close the K+ channels, slows the maximum action potential rate. Both for single action restoring the channel states to
Details
-
File Typepdf
-
Upload Time-
-
Content LanguagesEnglish
-
Upload UserAnonymous/Not logged-in
-
File Pages6 Page
-
File Size-