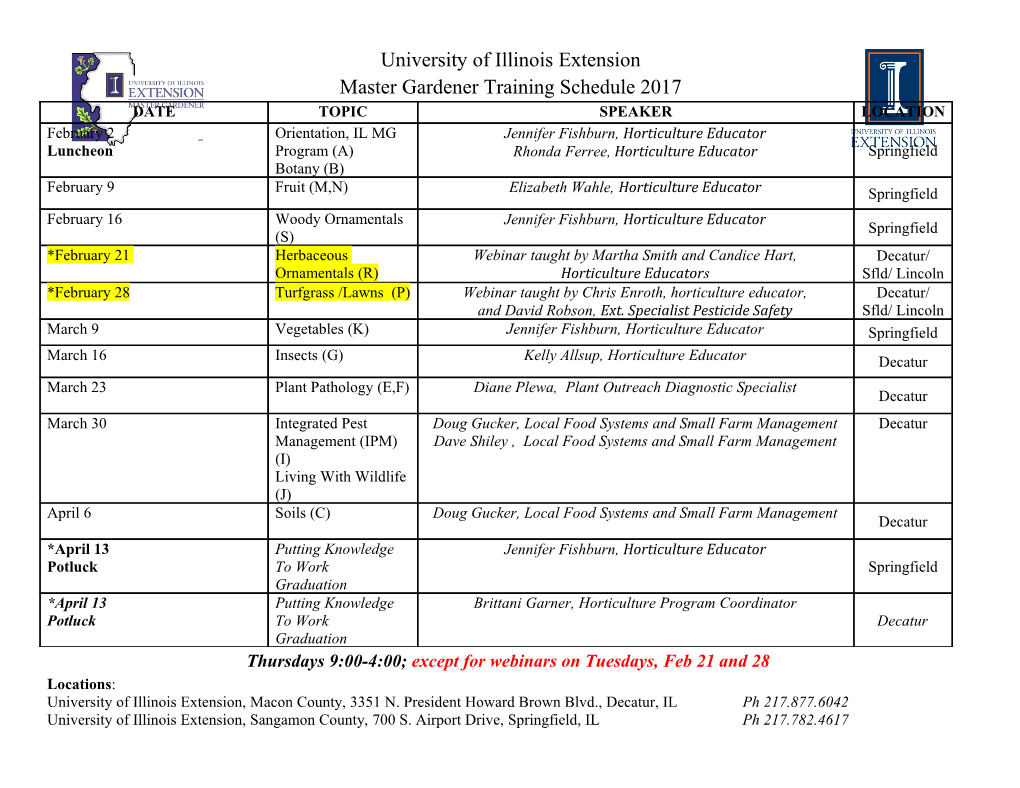
View metadata, citation and similar papers at core.ac.uk brought to you by CORE provided by University of Liverpool Repository Atom-at-a-time resonance ionization spectroscopy of nobelium Mustapha Laatiaoui1,2 , Werner Lauth3, Hartmut Backe3, Michael Block1,2,4, Dieter Ackermann2†, Bradley Cheal5, Prema- ditya Chhetri6, Christoph Emanuel Düllmann1,2,4, Piet Van Duppen7, Julia Even1†, Rafael Ferrer7, Francesca Giacoppo1,2, Stefan Götz2,4, Fritz Peter Heßberger1,2, Mark Huyse7, Oliver Kaleja2,8, Jadambaa Khuyagbaatar1,2, Peter Kunz9, Felix Lautenschläger6, Andrew Kishor Mistry1,2, Sebastian Raeder1,2,7, Enrique Minaya Ramirez1†, Thomas Walther6, Calvin Wraith5 & Alexander Yakushev2 1 Experimental study of atomic spectra tradition- 42 half-life of T1/2 = 20.1 h facilitated resonance laser ioniza- 11,12 2 ally required optical spectroscopy of a primordial 43 tion spectroscopy experiments . The transfermium ele- 3 isotope for each element, providing a benchmark 44 ments, in contrast, neither occur naturally on earth nor can 4 in understanding the atomic structure and reveal- 45 macroscopic samples be synthesized. These elements are 5 ing how relativistic effects increasingly impact the 46 typically produced at large accelerator facilities by heavy- 6 binding energy of orbital electrons of the heaviest 47 ion induced fusion-evaporation reactions, which only yield 7 elements. Such studies have been conducted for 48 low rates. Some of their atomic properties had been acces- 1 13,14 8 most elements and theoretical modelling can be 49 sible in aqueous-phase and gas-phase chemical studies . 2,3 9 performed to high precision . Today, no tabu- 50 Only recently, the first ionization potential was experimen- 10 lated spectral lines exist for the transfermium el- 51 tally determined for lawrencium (Z = 103, Lr) applying sur- 15 11 ements of an atomic number Z>100. These ra- 52 face ionization techniques . 12 dioactive elements are produced in nuclear fusion 53 Advancing optical studies to the region of the trans- 13 reactions at rates of a few atoms per second at 54 fermium elements calls for fast spectroscopy techniques of 14 most and must be studied ‘live’ immediately fol- 4 55 extreme sensitivity . Challenges in this research field in- 15 lowing their production, which so far precluded 56 clude producing such atoms, separating them from unde- 16 their optical spectroscopy. Here, we report on ra- 57 sired species, which are inevitably co-produced, manipu- 17 dioactive decay-detected resonance ionization spec- 58 lating and detecting them within seconds, to mention but 18 troscopy on the element nobelium (No), the 102nd 59 a few. In our experiments we applied a RAdiation De- 19 element in the periodic table, in which we identi- 60 tected Resonance Ionization Spectroscopy (RADRIS) tech- 1 1 20 fied the ground-state transition S → P . Com- 16,17 18 0 1 61 nique , using a two-step photoionization process for 21 bined with data from an observed Rydberg series, 62 ionizing nobelium atoms (Z = 102). Similar techniques had 22 an upper-limit for the ionization potential (IP) is 63 previously been employed for laser spectroscopy of short- 23 obtained. These accurate results provide a partic- 64 lived americium fission isomers at production rates as low 24 ular challenge for state-of-the-art relativistic many- 19,20 65 as ten per second . For such an approach to become fea- 5,6,7,8 25 body calculations addressing quantum electro- 66 sible for elements like nobelium, requires an extensive search 26 dynamic effects. The present work opens the door 67 for atomic transitions within a spectral range proposed by 27 for high-precision measurements of various atomic 68 state-of-the-art model calculations. Unambiguous identifi- 28 and nuclear properties also of elements heavier than 69 cation of the atoms is achieved via their unique radioactive 29 nobelium and motivates future theoretical work. 21 70 decay fingerprint . 254 30 Since the establishment of the actinide elements in the 71 The isotope No with a half-life T1/2 = 51.2 s was 31 periodic table, great efforts have been undertaken to in- 72 chosen for the first laser spectroscopy experiments. This 9 32 vestigate their atomic spectra . The prevailing strategy 73 isotope was produced in the two-neutron evaporation chan- 48 208 33 included deducing many of the atomic properties from a 74 nel of the complete-fusion reaction Ca+ Pb. The fusion 208 34 detailed knowledge of the electronic configuration, which 75 products, emerging from a thin Pb target, were separated 48 35 is subject to strong relativistic effects. This approach was 76 in-flight from the intense Ca primary beam by the Sepa- 22 36 driving large optical spectroscopy research programs for 77 rator for Heavy Ion reaction Products (SHIP) at the GSI 37 many years, which yielded detailed insight into the atomic 78 Helmholtzzentrum für Schwerionenforschung. About four 1 254 38 structure of elements up to einsteinium including precise 79 No ions per second were implanted in a buffer-gas stop- 4 39 values for their IP. The heaviest element for which optical 80 ping cell installed in the SHIP’s focal plane . They were 40 spectroscopy has hitherto been reported is fermium with 81 thermalized in 95 mbar high-purity argon gas and accumu- 255 41 Z = 100 (ref. 10). A sample of about 10 pg of Fm with a 82 lated and neutralized on a tantalum catcher filament (see 1Helmholtz-Institut Mainz, Johann-Joachim-Becher Weg 36, D-55128 Mainz, Germany. 2 GSI Helmholtzzentrum für Schwerionenforschung GmbH, Planckstr. 1, D-64291 Darmstadt, Germany. 3 Institut für Kernphysik, Johannes Gutenberg-Universität, Johann-Joachim-Becher-Weg 45, D-55128 Mainz, Germany. 4 Institut für Kernchemie, Johannes Gutenberg-Universität, Fritz-Strassmann-Weg 2, D-55128 Mainz, Germany. 5 Department of Physics, University of Liverpool, Oxford Street, L69 7ZE Liverpool, UK. 6 Institut für Angewandte Physik, Technische Universität Darmstadt, Schlossgartenstr. 7, D-64289 Darmstadt, Germany. 7 Instituut voor Kern- en Stralingsfysica, KU Leuven, Celestijnenlaan 200D, B-3001 Leuven, Belgium. 8 Institut für Kernphysik, Technische Universität Darmstadt, Schlossgartenstr. 9, D-64289 Darmstadt, Germany. 9 TRIUMF, 4004 Wesbrook Mall, Vancouver BC V6T 2A3, Canada. †Present addresses: Grand Accélérateur National d’Ions Lourds, Bd Henri Becquerel, BP 55027 - 14076 Caen Cedex 05, France (D.A.); KVI - Center for Advanced Radiation Technology, Rijksuniversiteit Groningen, Zernikelaan 25, 9747 AA Groningen, Netherlands (J.E.); Institut de Physique Nucléaire Orsay, 15 rue Georges Clémenceau, 91406 Orsay, France (E.M.R) 1 83 Methods section). For a short time during every measure- 141 sizable dipole-transition amplitude. We obtained a corre- 254 84 ment cycle, the incoming flux of No ions was turned off 142 sponding Einstein coefficient for spontaneous emission of +2.6 8 −1 85 and the adsorbed nobelium atoms were evaporated from the 143 Aki = 4.2−2.8 × 10 s by fitting a rate-equation model 86 filament by heating this briefly to a temperature of about 144 (P.C., H.B. et al., manuscript in preparation) to the satura- 14 2 1 87 1, 350 K. Nobelium atoms in the ground state 5f 7s S0 145 tion data. This value is in agreement with various theoret- 88 residing in laser beam paths in the vicinity of the filament 146 ical predictions (Table 1a) supporting that the short-lived 14 1 89 will undergo element-selective ionization in a two-step ex- 147 5f 7s7p P1 atomic state was observed. 90 citation scheme as shown in the inset of Fig. 1a. The ion- 148 We also observed several high-lying Rydberg states in 91 ization proceeded by resonantly exciting the singlet state 254 149 No (Fig. 3a-c). The first excitation step was set to the 14 1 92 5f 7s7p P1 with ultraviolet (UV) light from a tunable dye 0 −1 150 resonance at ν1 = 29, 961.457 cm , while the second step 93 laser (λ ), followed by a second excitation into the contin- −1 1 151 was scanned with a dye laser in the range from 23, 460 cm 94 uum beyond the IP with UV light in the wavelength range −1 152 to 23, 503 cm . Two pronounced peaks, potentially mem- 95 349-353 nm (λ ) from a more powerful excimer laser. In- 2 153 bers of the same Rydberg series, were observed. Sampling 96 duced photoions are subsequently guided by electrostatic 154 in an extended scan range revealed further peaks belong- 97 fields to a silicon detector where the characteristic alpha 155 ing to the same series, which so far could not be assigned 254 23 98 decay of No is detected . 156 unambiguously. −1 99 A spectral range from 28, 887 to 33, 191 cm was 1 1 100 chosen to locate the S → P atomic transition 0 1 a 101 in nobelium (Methods). Latest predictions based on 0.6 102 Multi-Configuration-Dirac-Fock (MCDF) and Relativistic- ) IP 1 5,6,7,8 - 103 Coupled-Cluster calculations suggest wavenumber val- 2, l2 0.4 254 1 −1 No P1 104 ues clustering between 29, 256 and 31, 709 cm (Table 1a) 1 1, l1 105 for the excited state P1. The sufficient treatment of cor- ) -1 1 106 relation effects by the different approaches decisively im- 0.2 S0 24 107 proved the picture given by the first MCDF calculations 108 focusing on quantum electrodynamic effects. Nonetheless, 0.0 109 about 6, 110 laser scan steps needed to be conducted be- b 0.06 110 fore discovering this elusive first-step resonance. In Fig. 1a (s rate Count ) 1 - 111 (full triangles) we show the observed resonance in terms of 252No Count rate (s Count 112 normalized alpha-decay count rates.
Details
-
File Typepdf
-
Upload Time-
-
Content LanguagesEnglish
-
Upload UserAnonymous/Not logged-in
-
File Pages10 Page
-
File Size-