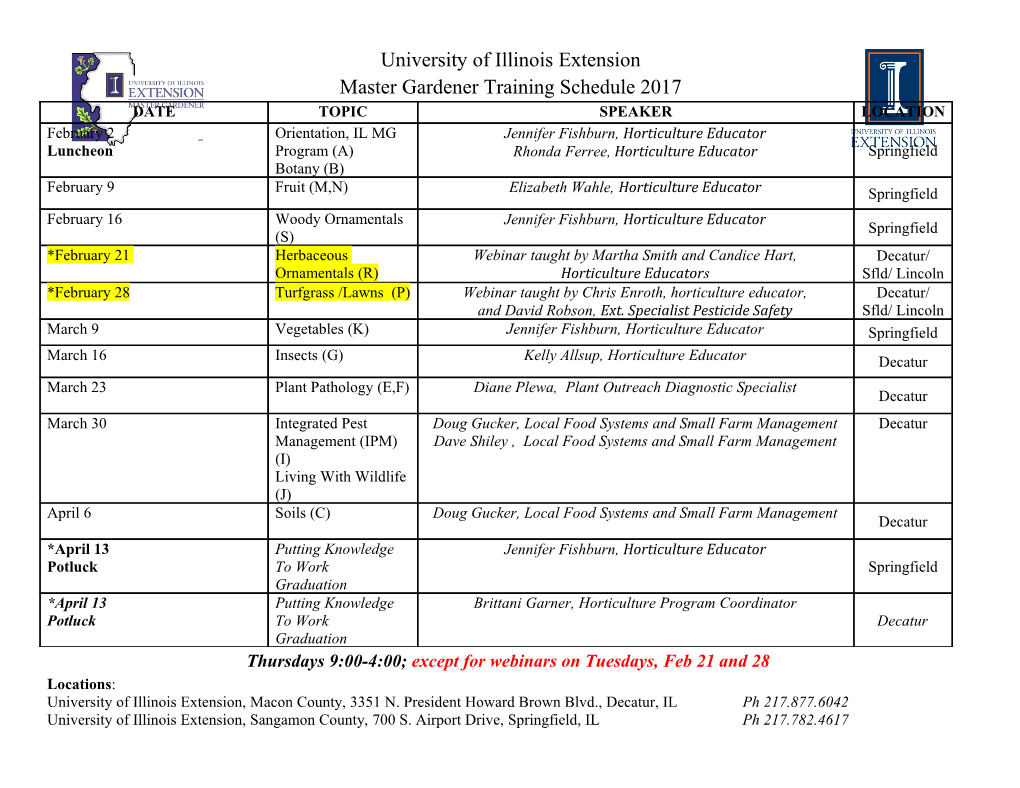
Downloaded from genesdev.cshlp.org on September 24, 2021 - Published by Cold Spring Harbor Laboratory Press REVIEW Intricate SUMO-based control of the homologous recombination machinery Nalini Dhingra and Xiaolan Zhao Molecular Biology Department, Memorial Sloan Kettering Cancer Center, New York, New York 10065, USA The homologous recombination (HR) machinery plays regulation in mammalian cells and highlight their simi- multiple roles in genome maintenance. Best studied in larities and differences with those found in yeast. It is the context of DNA double-stranded break (DSB) repair, noteworthy that SUMO plays important roles in modulat- recombination enzymes can cleave, pair, and unwind ing protein recruitment to damaged chromatin and in oth- DNA molecules, and collaborate with regulatory proteins er DNA break repair pathways. As these topics have been to execute multiple DNA processing steps before generat- well covered in other reviews (Schwertman et al. 2016; ing specific repair products. HR proteins also help to cope Garvin and Morris 2017), they are not addressed here in or- with problems arising from DNA replication, modulating der to maintain the focus on the regulation of core HR impaired replication forks or filling DNA gaps. Given machinery. these important roles, it is not surprising that each HR step is subject to complex regulation to adjust repair effi- ciency and outcomes as well as to limit toxic intermedi- Overview of the SUMO pathway and the effects ates. Recent studies have revealed intricate regulation of of sumoylation all steps of HR by the protein modifier SUMO, which SUMO, a small protein of ∼100 residues, is highly con- has been increasingly recognized for its broad influence served among eukaryotes with several isoforms found in in nuclear functions. This review aims to connect estab- mammalian cells. SUMO can be conjugated to the lysine lished roles of SUMO with its newly identified effects residues of target proteins via the action of SUMO E1, on recombinational repair and stimulate further thought E2, and E3 enzymes (Fig. 1; Johnson 2004). The SUMO on many unanswered questions. E1 helps to covalently link SUMO to the E2 enzyme’s ac- tive site. In many cases, SUMO E2 can directly bind to the sumoylation consensus sequence ψ-K-X-E/D (ψ: hydropho- Homologous recombination is critical for several aspects bic residue) or its reverse sequence on the target proteins, of life, ranging from DNA repair and genome duplication permitting the transfer of SUMO to the lysine residue (Ro- to gamete production. Our understanding of HR pathways driguez et al. 2001; Sampson et al. 2001). However, in vivo has benefited from a combination of assay systems. In sumoylation often requires SUMO E3s, which promote cells, the generation of a defined DSB allows quantitative productive configurations for SUMO transfer by simulta- assessment of the status of the broken DNA molecules neously binding the SUMO-charged E2 and the substrate and the repair proteins at a temporal resolution, as well (Werner et al. 2012; Streich and Lima 2016). Organisms as determination of the genetic requirement for each examined so far contain a single SUMO E1 and E2 but mul- step of repair (Haber 2016). Extensive biochemical analy- tiple SUMO E3s to confer some levels of substrate specif- ses and more recently single molecule experiments have icity. However, substrate overlap is also seen for SUMO further defined the activities of HR enzymes and elucidat- E3s. Such redundancy can increase the probability of ed how they can collaborate in multiple HR steps. Several sumoylation and thus the robustness of SUMO-based reg- recent reviews have discussed these findings in detail (Sy- ulation. Earlier genetic studies have implicated sumoyla- mington et al. 2014; Heyer 2015; Ranjha et al. 2018); thus, tion enzymes in the regulation of HR and genome we give only a brief overview here for each HR step to pro- stability from yeast to humans (Xhemalce et al. 2004; vide the context of SUMO-based regulation. As the HR Zhao and Blobel 2005; Branzei et al. 2006; Galanty et al. machinery and its sumoylation are best examined in bud- 2009; Morris et al. 2009). Further examination of how ding yeast, we use this system as an index for summariz- sumoylation affects substrate properties has begun to ing SUMO-based control. We also discuss additional shed light on the basis of SUMO-mediated regulation. © 2019 Dhingra and Zhao This article is distributed exclusively by Cold [Keywords: double-strand break repair; genome maintenance; Spring Harbor Laboratory Press for the first six months after the full-issue homologous recombination; sumoylation] publication date (see http://genesdev.cshlp.org/site/misc/terms.xhtml). Corresponding author: [email protected] After six months, it is available under a Creative Commons License (Attri- Article is online at http://www.genesdev.org/cgi/doi/10.1101/gad.328534. bution-NonCommercial 4.0 International), as described at http://creative- 119. commons.org/licenses/by-nc/4.0/. 1346 GENES & DEVELOPMENT 33:1346–1354 Published by Cold Spring Harbor Laboratory Press; ISSN 0890-9369/19; www.genesdev.org Downloaded from genesdev.cshlp.org on September 24, 2021 - Published by Cold Spring Harbor Laboratory Press Role of sumoylation in homologous recombination SUMO E1 + S teins from chromatin or from protein association is medi- (Aos1/Uba2) ated by the Cdc48 segregase (Torrecilla et al. 2017). In both budding and fission yeasts, the Cdc48 cofactor SUMO E2–– S (Ubc9) Ufd1 uses its SIM sequences to recognize sumoylated pro- SUMO E3s teins, targeting the segregase to specific substrates for (Siz1, Siz2, Mms21) their extraction and sometimes degradation (Bergink Desumoylases Alter protein functions et al. 2013; Kohler et al. 2013). Collectively, STUbLs, (Ulp1, Ulp2) S (e.g. regulate interactions with Uls1 family proteins, and the Cdc48 segregase provide Substrate Substrate protein or DNA, change protein stability, localization, or activity) multiple avenues to regulate the fates of the SUMO tar- Uls1 Cdc48 Slx8 gets (Fig. 1). Competing with these accessary factors are the SUMO- Substrate removal Substrate removal Substrate degradation from DNA or recycle from DNA or degradation DNA lesion relocalization specific proteases or desumoylases that can cleave SUMO off these proteins or collapse SUMO chains (Fig. 1; Hickey et al. 2012). The distinct cellular localization of these Figure 1. A simplified scheme for the SUMO pathway and con- sequences of sumoylation. Sumoylation of a substrate requires desumoylases provides an important strategy to diversify the sequential action of SUMO E1, E2, and E3 enzymes. The their substrate repertoire. The multiple means to remove SUMO moiety (S) covalently linked to the substrates can modu- SUMO from proteins or degrade sumoylated proteins late protein functions in different ways (see text for detail). Rever- attest to the highly dynamic nature of cellular sumoyla- sal of sumoylation is catalyzed by the desumoylases. The yeast tion, and the difficulty of detecting the low levels of SUMO pathway enzymes are indicated. In some contexts, a sumoylated forms. In addition, the so-called “on-site” sumoylated substrate can be recognized by SUMO pathway acces- sumoylation, wherein DNA metabolism factors are only sory factors, as represented by the budding yeast proteins Uls1, sumoylated once they have engaged with DNA for specif- Cdc48, and Slx5/8. These accessory proteins can regulate the fates ic transactions, can also explain the low levels of sumoy- of sumoylated proteins in distinct manners as detailed in the text. lated forms detected in vivo (Sarangi and Zhao 2015). It is important to comprehend how the SUMO forms of sub- Collective evidence suggests that the SUMO moiety strates can exert specific effects before their elimination can change substrate attributes in multiple ways, includ- by the factors described above. Although multiple chal- ing altering its activities or interactions with other bio- lenging issues remain to be addressed, studies of the molecules (Flotho and Melchior 2013; Pichler et al. sumoylation of HR proteins have provided insights into 2017). The diversity of SUMO’s effects is described below this question as summarized below. in the context of each HR step. It is worthwhile to high- light a prevalent effect of sumoylation that is mediated by binding to SUMO interaction motifs (SIMs) composed DNA end resection factors, their roles in sumoylation, of a hydrophobic core preceded or followed by negatively and their regulation by SUMO charged residues (Song et al. 2004; Hecker et al. 2006). The SUMO:SIM interaction is relatively weak, a feature The canonical HR pathway that repairs DSBs can be rough- that can actually be useful for modulating dynamic pro- ly divided into three stages that are conserved from yeast cesses involving many protein hand-over events such as to humans. In the first stage, the DNA end resection ma- HR. Indeed, multiple HR factors contain SIMs, some of chinery generates 3′ single strand DNA (ssDNA) to be which have been shown to promote interactions with used for homology searching (Symington 2016). In budding sumoylated proteins while others await further examina- yeast, DNA end resection involves the initial trimming of tion (detailed below). The SUMO:SIM interaction can also 5′ DNA ends by the MRX (Mre11–Rad50–Xrs2) nuclease in aid more stable association if additional binding interfaces collaboration with Sae2, which is followed by extended are present. In some instances, stronger interactions are DNA degradation by either the helicase-nuclease duo established when several SIMs from a protein bind to mul- Sgs1 (with cofactor Top3-Rmi1) and Dna2, or the exonu- tiple SUMO moieties conjugated to the substrates or to clease Exo1 (Fig. 2; Symington 2016). SUMO itself (SUMO chains). The resultant ssDNA is bound by the ssDNA-binding A family of multi-SIM containing proteins, STUbLs complex RPA (Rfa1–3) to prevent secondary structure for- (SUMO targeted ubiquitin ligases), have a wide-ranging mation or DNA degradation.
Details
-
File Typepdf
-
Upload Time-
-
Content LanguagesEnglish
-
Upload UserAnonymous/Not logged-in
-
File Pages10 Page
-
File Size-