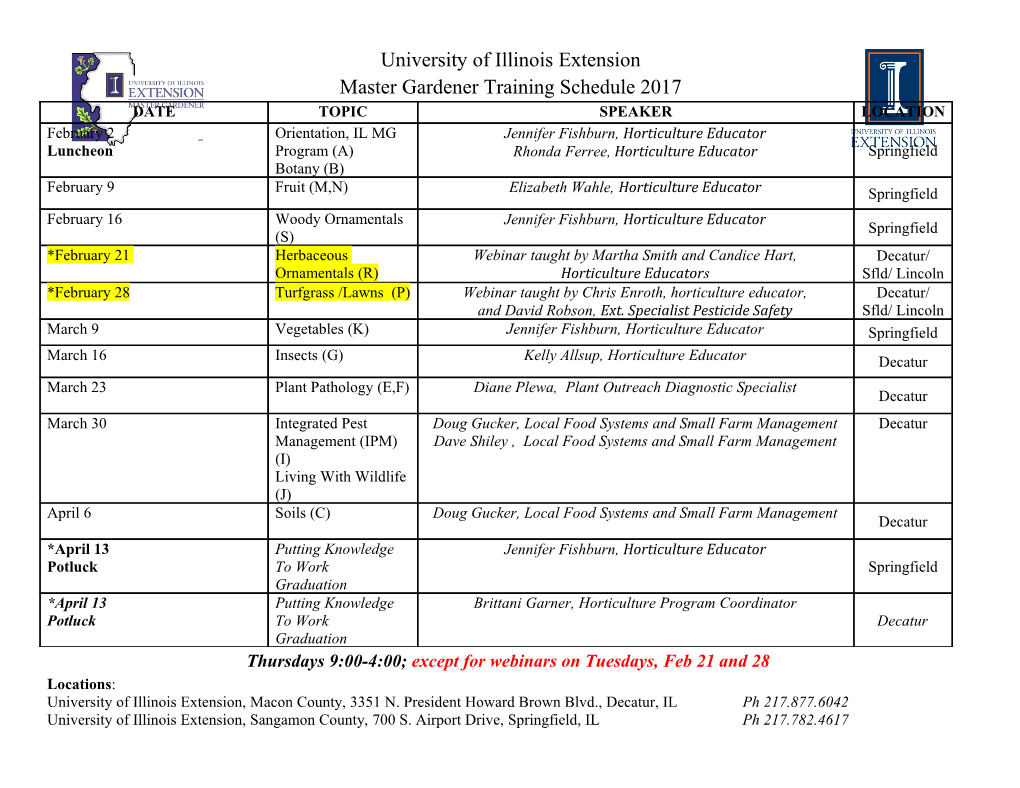
Life 2014, 4, 281-294; doi:10.3390/life4030281 OPEN ACCESS life ISSN 2075-1729 www.mdpi.com/journal/life Review Cognitive Neuroscience in Space Gabriel G. De la Torre Department of Psychology, University of Cadiz, Campus Río San Pedro S/N, 11510 Puerto Real (Cadiz), Spain; E-Mail: [email protected]; Tel.: +34-646-287398 Received: 19 March 2014; in revised form: 11 June 2014 / Accepted: 23 June 2014 / Published: 3 July 2014 Abstract: Humans are the most adaptable species on this planet, able to live in vastly different environments on Earth. Space represents the ultimate frontier and a true challenge to human adaptive capabilities. As a group, astronauts and cosmonauts are selected for their ability to work in the highly perilous environment of space, giving their best. Terrestrial research has shown that human cognitive and perceptual motor performances deteriorate under stress. We would expect to observe these effects in space, which currently represents an exceptionally stressful environment for humans. Understanding the neurocognitive and neuropsychological parameters influencing space flight is of high relevance to neuroscientists, as well as psychologists. Many of the environmental characteristics specific to space missions, some of which are also present in space flight simulations, may affect neurocognitive performance. Previous work in space has shown that various psychomotor functions degrade during space flight, including central postural functions, the speed and accuracy of aimed movements, internal timekeeping, attentional processes, sensing of limb position and the central management of concurrent tasks. Other factors that might affect neurocognitive performance in space are illness, injury, toxic exposure, decompression accidents, medication side effects and excessive exposure to radiation. Different tools have been developed to assess and counteract these deficits and problems, including computerized tests and physical exercise devices. It is yet unknown how the brain will adapt to long-term space travel to the asteroids, Mars and beyond. This work represents a comprehensive review of the current knowledge and future challenges of cognitive neuroscience in space from simulations and analog missions to low Earth orbit and beyond. Keywords: cognitive neuroscience; space psychology; human space flight; human factors Life 2014, 4 282 1. Introduction The human brain evolved very rapidly after our ancestors began to stand up and walk. This standing position favored the use of hands and tools, a key aspect in the ongoing human evolutionary process. Our brain has the ability to continuously adapt to new situations and demands from our environment. Like children progressing through their developmental stages, astronauts have to adapt to a completely new and weightless environment. Balance, movement and other brain functions are affected under zero gravity or microgravity conditions. Space cognitive neuroscience seeks to understand how the brain and mind react to the special environmental conditions of space. Among the factors inherent to the space environment that may affect the human brain and mind are microgravity, radiation, weightlessness, acceleration, noise and stress. The study of the human brain in space has become an important subject in recent years. The first studies on space neuroscience go back to 1962 during the Russian Vostok-3 mission, when some sensory-motor studies were carried out. On Earth, new brain imaging techniques, neuropsychological assessment tools and other physiological measures have been developed to enable very detailed studies of brain activity and cognitive functioning. For neuroscientists, as well as psychologists, it is of high relevance to understand the underlying neurocognitive and neuropsychological parameters of space flight. Unfortunately, standard brain imaging techniques (e.g., functional magnetic resonance imaging (fMRI)) are not applicable in space, due to the payload restrictions of space missions and costs. The European module ―Columbus‖, a part of the International Space Station (ISS), was equipped with an electroencephalography (EEG) system as a tool to research the link between weightlessness and central nervous system (CNS) activity [1]. Neurocognitive tests, electrophysiological measurements and other related methods are commonly used in space to assess brain activity, neurocognitive and behavioral status and the mental health of astronauts. Earth-based research may also help to further our understanding of how the brain functions in space. This is achieved using so-called space analog environments and space simulations; both can be defined by their extreme environmental characteristics. The most well-known space analogs are the Antarctic and Arctic stations and desert and submarine installations. At these facilities, crewmembers spend months in isolation and harsh weather conditions performing a variety of tasks and procedures similar to those carried out in space missions. Other research paradigms that help in the field of space neuroscience include bed-rest experiments. When astronauts return from a long flight, their bodies need days to recuperate from the effects of living in weightlessness. In bed-rest experiments, the subjects remain in supine position on a bed for long periods, with the head titled slightly downwards while performing different tasks. Bed-rest studies resemble some aspects of weightlessness, allowing scientists to probe how the body reacts to microgravity and to test methods for keeping future astronauts fit and healthy. A well-known threat to the success of space missions is the inadequate and ineffective performance of the crew. Results from Earth-based research highlight the importance of studying the effects of stress on cognitive performance. Cognitive and perceptual motor performances deteriorate under stress [2–4]. We can thus expect similar effects in the stressful environment of a space mission and in extreme environments and simulations. Previous work has shown that various psychomotor functions are degraded during space flight, among them central postural functions [5,6] involving Life 2014, 4 283 hierarchically organized brain areas, including motor cortex in frontal lobes, basal ganglia, vestibular system in the midbrain and cerebellum, the speed [7–9] and accuracy of aimed movements [5,10] associated, among others, with primary motor cortex, cerebellum and visual cortex, internal timekeeping [11] related to prefrontal cortex and striatum, attentional processes [12] distributed in different brain areas, such as frontal and parietal cortex, superior colliculi subcortical region, frontal eye field and anterior cingulate cortex, limb position sense [13,14], including the primary somatosensory cortex and cerebellum, and the central management of concurrent tasks [15] involving mainly prefrontal, temporal and parietal cortex and basal ganglia (Figure 1). Such psychomotor deficits have been implicated as the causes of accidents in space [16]. It remains unknown to what extent these observed deficits might change during long-term space missions, as on a flight to Mars, for example. Results from Mars-500, one of the longest space mission simulations ever, revealed that a variety of neurological and psychological factors, such as circadian rhythms and social behavior, were affected by characteristics of the mission and the special environment [17]. Figure 1. Cortical areas of the human brain affected in space. Numbers used for anatomical reference correspond to Brodmann areas. 1, 2 and 3: primary somatosensory cortex; 4: primary motor cortex; 5: somatosensory association area; 6: premotor cortex; 7: parietal cortex; 8: frontal eye field; 10, 11, 47: prefrontal cortex; 17, 18: visual cortex; 22: auditory cortex; 34: dorsal entorhinal cortex. 2. Neuroscience in Space 2.1. Microgravity and Space Motion Sickness Gravity has shaped life on Earth. It is perceived by all organisms, from unicellular forms to humans, determines our orientation in space and helps control posture. We have specialized organs, such as the vestibular system in the inner ear, for gravity perception. Sensory information about motion, equilibrium and spatial orientation is provided by the vestibular apparatus in each ear, which includes the utricle, saccule and three semicircular canals. The utricle and saccule detect gravity (vertical Life 2014, 4 284 orientation) and linear movement. The semicircular canals, which detect rotational movement, are located at right angles to each other and are filled with a fluid called endolymph. When the head rotates, the direction is sensed by a particular canal. The endolymphatic fluid within the canal lags behind, due to inertia, and exerts pressure against the canal’s sensory receptors. The receptors then send impulses to the brain conveying information about movement. When the vestibular organs on both sides of the head are functioning properly, they send symmetrical impulses to the brain (Figure 2). Figure 2. The vestibular system. Balance information provided by the peripheral sensory organs—eyes, muscles and joints and the two sides of the vestibular system—is relayed to the brain stem. There, it is processed and integrated with learned information contributed by the cerebellum (the coordination center of the brain) and the cerebral cortex (the thinking and memory center). A person can become disoriented if the sensory input received from his or her eyes, muscles and joints, or vestibular organs conflict with one another,
Details
-
File Typepdf
-
Upload Time-
-
Content LanguagesEnglish
-
Upload UserAnonymous/Not logged-in
-
File Pages14 Page
-
File Size-