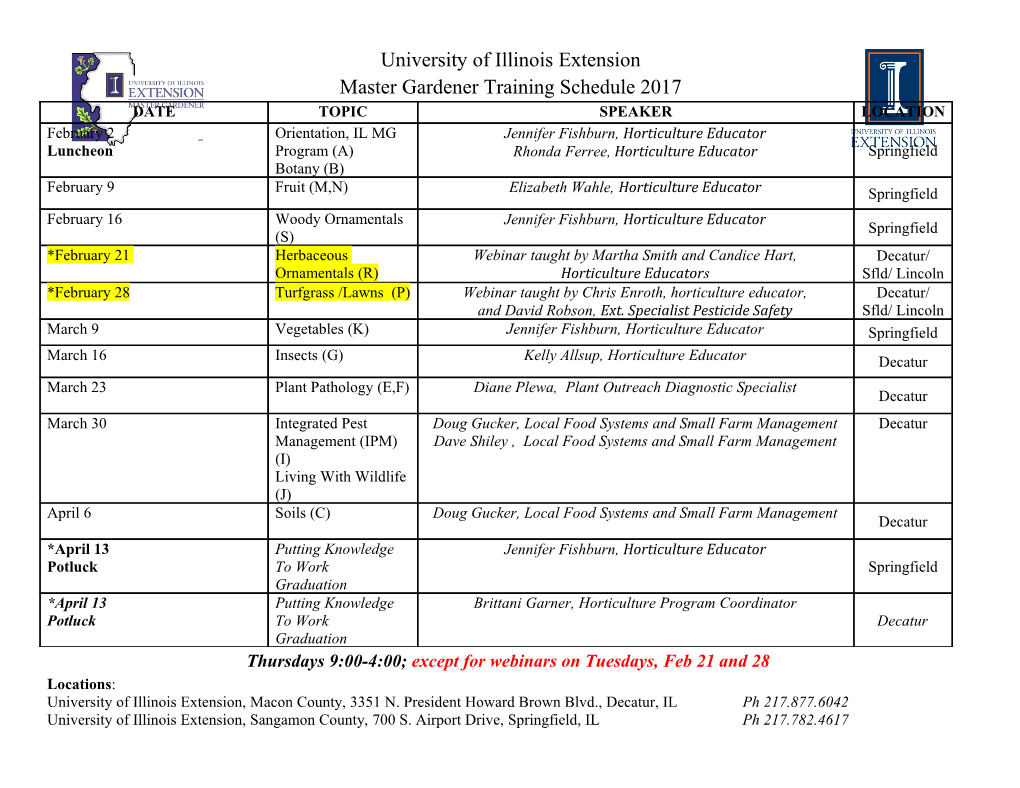
Cristina Japu Cyclic derivatives of D-glucose and tartaric acid as building blocks for renewable polyesters Doctoral Thesis Supervised by: Prof. Sebastián Muñoz Guerra Dr. Antxon Martínez de Ilarduya Barcelona, 2014 Departament d’Enginyeria Química Escola Tècnica Superior d’Enginyeria Industrial de Barcelona (ETSEIB) Universitat Politècnica de Catalunya (UPC) Table of content Chapter 1. Aim and outline of this Thesis Chapter 2. The chemistry and the applications of polyesters 2.1. Introduction 2.2. Green monomers 2.2.1. Bio-based routes for some conventional monomers 2.2.2. Carbohydrate-based monomers as building blocks for polyesters 2.3. Aromatic polyesters 2.4. References PART I Copolyesters derived from tartaric acid Chapter 3. Biobased poly(hexamethylene terephthalate) copolyesters containing cyclic acetalized tartrate units 3.1. Introduction 3.2. Experimental section 3.2.1. Materials and methods 3.2.2. Synthesis of monomers 3.2.3. Synthesis of polyesters 3.2.4. Hydrolytic and enzymatic degradation assays 3.3. Results and discussion 3.3.1. Polyesters synthesis and microstructure of PHTxThxy copolyesters 3.3.2. Thermal properties 3.3.3. Structure and isothermal crystallization 3.3.4. Hydrolytic and enzymatic degradability 3.4. Conclusions 3.5. References Supporting information Chapter 4. Bio-based poly(ethylene terephthalate) copolyesters made from cyclic monomers derived from tartaric acid 4.1. Introduction 4.2. Experimental section 4.2.1. Materials and methods 4.2.2. Synthesis of monomers 4.2.3. Synthesis of polyesters and 4.3. Results and discussion 4.3.1. Polyesters synthesis 4.3.2. Microstructure of PExThxyT and PETxThxy copolyesters 4.3.2. Thermal properties 4.3.3. Structure and isothermal crystallization 4.4. Conclusions 4.5. References Supporting information PART II Copolyesters derived from D-glucose Chapter 5. Bio-based aromatic copolyesters made from 1,6-hexanediol and bicyclic diacetalized D-glucitol 5.1. Introduction 5.2. Experimental section 5.2.1. Materials and methods 5.2.2. Synthesis of monomers 5.2.3. Synthesis of polyesters 5.2.4. Hydrolytic and enzymatic degradation assays 5.3. Results and discussion 5.3.1. Polyesters synthesis and microstructure of PHxGluxyT copolyesters 5.3.2. Thermal properties 5.3.3. Structure and isothermal crystallization 5.3.4. Hydrolytic and enzymatic degradability 5.4. Conclusions 5.5. References Supporting information Chapter 6. D-Glucose-derived PET copolyesters with enhanced Tg 6.1. Introduction 6.2. Experimental section 6.2.1. Materials and methods 6.2.2. Synthesis of monomers 6.2.3. Synthesis of polyesters 6.2.4. Hydrolytic and enzymatic degradation assays 6.3. Results and discussion 6.3.1. Polyesters synthesis and microstructure of PExGluxyT copolyesters 6.3.2. Thermal properties 6.3.3. Structure, isothermal crystallization and stress-strain behaviour 6.3.4. Hydrolytic and enzymatic degradability 6.4. Conclusions 6.5. References Supporting information Chapter 7. Bio-based PBT copolyesters derived from D-glucose: influence of composition on properties 7.1. Introduction 7.2. Experimental section 7.2.1. Materials and methods 7.2.2. Synthesis of polyesters 7.2.4. Hydrolytic and enzymatic degradation assays 7.3. Results and discussion 7.3.1. Polyesters synthesis 7.3.2. Microstructure of PBxGluxyT and PBTxGluxy copolyesters 7.3.3. Thermal properties 7.3.4. Isothermal crystallization 7.3.5. X-ray diffraction and stress-strain behaviour 7.3.6. Hydrolytic and enzymatic degradability 7.4. Conclusions 7.5. References Supporting information Chapter 8. Biodegradable copolyesters of poly(hexamethylene terephthalate) containing bicyclic 2,4:3,5-di-O-methylene-D-glucarate units 8.1. Introduction 8.2. Experimental section 8.2.1. Materials and methods 8.2.2. Synthesis of polyesters 8.2.3. Hydrolytic and enzymatic assays 8.3. Results and discussion 8.3.1. Polyesters synthesis and microstructure of PHTxGluxy copolyesters 8.3.2. Thermal properties and X-ray diffraction 8.3.3. Hydrolytic and enzymatic degradability 8.4. Conclusions 8.5. References Supporting information Chapter 9. Sustainable copolyesters made from 1,4-butanediol, sebacic acid and D- glucose by melt and enzymatic polycondensation 9.1. Introduction 9.2. Experimental section 9.2.1. Materials and methods 9.2.2. Synthesis of polyesters by melt polycondensation 9.2.3. Synthesis of polyesters by enzymatic polycondensation 9.2.4. Hydrolytic and enzymatic assays 9.3. Results and discussion 9.3.1. Melt polycondensation-synthesis and microstructure 9.3.2. Synthesis with Candida antarctica 9.3.3. Thermal properties and X-ray diffraction 9.3.4. Biodegradation and hydrolytic degradability 9.4. Conclusions 9.5. References Supporting information Chapter 10. General conclusions Patents and Publications derived from the Thesis Conference proceedings Acknowledgements Glossary MeOH Methanol Mn Number-average molecular weight Mw Weight-average molecular weight n.d. Not determined NMR Nuclear magnetic resonance PBS Poly(butylene succinate) PBSeb Poly(butylene sebacate) PCL Poly(-caprolactone) PET Poly(ethylene terephthalate) PHA Polyhydroxyalkanoate PHB Polyhydroxybutyrate PLA Poly(lactic acid) PMMA Poly(methyl methacrylate) POM Polarizing optical microscopy σ Tensile strength SEM Scanning electron microscopy SI Supporting information Tc Crystallization temperature Td Temperature for maximum decomposition rate (in TGA) TFA Trifluoroacetic acid Tg Glass transition temperature TGA Termogravimetric analysis Tm Melting temperature TMS Tetramethylsilane Wavenumber (cm-1) W Remaining final weight (in TGA) WAXS Wide angle X-ray scattering XRD X-Ray diffraction ΔHc Crystallization enthalpy ΔHm Melting enthalpy Chapter 1. Aim and outline of this Thesis Aim and outline of this Thesis Aim and outline of this Thesis Polymers play a prominent role as extraordinarily versatile and diversified structural and multifunctional macromolecular materials. Without polymers, modern life would be impossible because polymers assure the high quality of life with the development of modern technologies. The growing environmental awareness over limited fossil fuel reserves (and consequently the increase of oil prices) has stimulated the search for novel polymeric materials and production processes drawn from renewable feedstocks. In addition, from the widespread use of synthetic polymeric materials has emerged another major concern; indeed, despite the increasing popularity of plastic recycling, disposal of these undegradable materials has led to serious environmental pollution. From this point of view, the continuous depletion of landfill space available for discarded plastic wastes leads to the need for biodegradable polymeric materials to be used as substitutes for non-degradable conventional plastics. Such biocompatible and biodegradable polymers are thus currently emerging as valuable alternatives to conventional synthetic (petroleum-based) polymers and might be produced from raw materials. Although this renewed interest in polymers from renewable resources has gained wings recently, this whole idea is not new. Indeed, the advent of bio-based polymeric materials is entangled with the birth of macromolecular science and technology in the 19th century with the synthesis of some very well- known materials such as celluloid, vulcanized natural rubber or even nylon-6,6. At the beginning of the 21st Century, we are experiencing a renaissance of renewable polymers and a major thrust towards the development of bio-based macromolecular materials. Actually, the production of bio-plastics (partially or totally bio-based, biodegradable or both) is already a growing niche market that has increased from less than 300 000 metric tons in 2009 to surpass 1.0 million metric tons in 2011 and with a forecast of 5.8 million metric tons for 2016. Fermentation of glucose, obtained from lignocelluloses and starch, can be used to produce a great variety of bio-based monomers. For example, bioethanol is readily converted into ethylene, propylene, and also butadiene that can be used to synthesize bio-based polyolefins and butadiene rubber. Also available are diols, polyols, diacids, hydroxyalkanoic acids, aminoalkanoic acids, and diamines that can be used in melt polycondensation reactions to produce bio-based polyesters, polyamides, polycarbonates, as well as thermoset resins such as unsaturated polyesters and air-drying finishes. Starting from glucose, it is possible to obtain sorbitol, isosorbide (Is), and also a large variety of furans, most of which are not natural products. Furan derivatives offer attractive opportunities for preparing bio-based thermoset and thermoplastic resins from furfuryl alcohol, furfuryl aldehyde, hydroxymethyl furfuryl aldehyde, and 2,5- furandicarboxylic acid (FDCA). Side-products of biofuel production, such as glycerol, are also attractive bioresources for producing bio-based monomers such as acrylic acids, epichlorohydrine, 1,3-propanediol, and acrolein. 3 Chapter 1 The synthesis of polyesters and copolyesters made from monomers obtained by chemical modification of naturally-occurring compounds may lead to renewable polyesters with improved properties regarding to those displayed by the traditional ones. Carbohydrates are suitable resources to provide bio-based building block monomers useful for polycondensation. For example FDCA appears to be an excellent replacement for terephthalic acid in the production of aromatic polyesters. Another example is the incorporation of rigid diols such as isosorbide in polyesters was shown to increase glass transition
Details
-
File Typepdf
-
Upload Time-
-
Content LanguagesEnglish
-
Upload UserAnonymous/Not logged-in
-
File Pages278 Page
-
File Size-