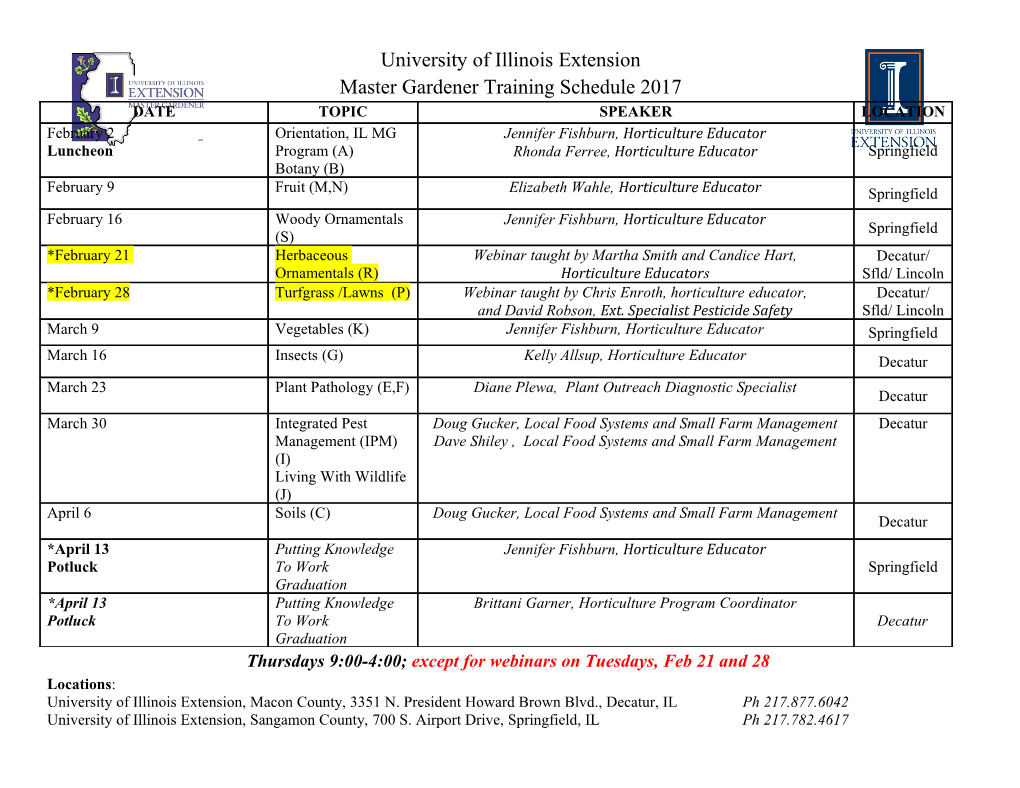
Topology: 2020, SEU Yi Li SCHOOL OF MATHEMATICS AND SHING-TUNG YAU CENTER,SOUTHEAST UNIVERSITY,NANJING,CHINA 210096 E-mail address: [email protected]; [email protected]; [email protected] Contents Chapter 1. Basic topology 1 1.1. Metric spaces 1 1.2. Topological spaces 11 1.3. Constructions 37 1.4. Topological groups and matrix Lie groups 50 1.5. Connectedness and compactness 67 Chapter 2. Analysis on topological groups 81 2.1. Haar measures 81 2.2. Iwasawa’s decomposition 87 2.3. Harish, Mellin and spherical transforms 92 Chapter 3. Fundamental groups 109 v CHAPTER 1 Basic topology Main references: • Kelley, John L. General topology, Reprint of the 1955 edition [Van Nostrand, Toronto, Ont.], Graduate Texts in Mathe- matics, No. 27, Springer-Verlag, New York-Berlin, 1975. xiv+298 pp. MR0370454 (51# 6681) • Bredon, Glen E. Topology and geometry, Graduate Texts in Mathematics, No. 139, Springer-Verlag, New York, 1993. xiv+557 pp. MR1224675 (94d: 55001) • Lee, John M. Introduction to topological manifolds, Second edi- tion, Graduate Texts in Mathematics, No. 202, Springer, New York, 2011. xviii+433 pp. ISBN: 978-1-4419-7939-1 MR2766102 (2011i: 57001) • Munkres, James R. Topology: a first course, Prentice-Hall, Inc., Englewood Cliffs, N. J., 1975. xvi+413 pp. MR0464128 (57 # 4063) • Burago, Dmitri; Burago, Yuri; Ivanov, Sergei. A course in metric geometry, Graduate Studies in Mathematics, 33, Amer- ican Mathematical Society, Providence, RI, 2001. xiv+415 pp. ISBN: 0-8218-2129-6 MR1835418 (2002e: 53053) 1.1. Metric spaces 1.1.1. Metric spaces. A metric on a set X is a map d : X × X ! R := R [ f¥g satisfying, for any x, y, z 2 X, (a) (positiveness) d(x, y) ≥ 0 and d(x, y) = 0 if and only if x = y; (b) (symmetry) d(x, y) = d(y, x); (c) (triangle inequality) d(x, z) ≤ d(x, y) + d(y, z). A metric space is a pair (X, d) where d is a metric on X. Given a metric space (X, d) we define a relation ∼ on X by x ∼ y () d(x, y) < ¥. Then ∼ is an equivalence relation on X, and the equivalence class [x] of x can be endowed with a natural metric (still denoted by d). EXERCISE 1.1.1. For any x 2 X, show that ([x], d) is a finite metric space. ! A map f : (X, dX) (Y, dY) between metric spaces is called distance-preserving if dY( f (x1), f (x2)) = dX(x1, x2) 1 2 1. BASIC TOPOLOGY 2 for all x1, x2 X. A distance-preserving bijection is called an isometry. A semi-metric on a set X is a map d : X × X ! R := R [ f¥g satisfying, for any x, y, z 2 X, (a) (nonnegativity) d(x, y) ≥ 0; (b) (symmetry) d(x, y) = d(y, x); (c) (triangle inequality) d(x, z) ≤ d(x, y) + d(y, z). A semi-metric space is a pair (X, d) where d is a semi-metric on X. Given a semi-metric space (X, d) we define a relation ∼ on X by x ∼ y () d(x, y) = 0. Then ∼ is an equivalence relation on X. Define Xˆ := X/ ∼= f[x] : x 2 Xg, dˆ([x], [y]) := d(x, y). Then (X/d, d) := (Xˆ , dˆ) is a metric space. EXERCISE 1.1.2. Show that dˆ is well-defined and (X/d, d) is a metric space. EXERCISE 1.1.3. Let X = R2 and define 0 0 0 0 d((x, y), (x , y )) := j(x − x ) + (y − y )j. Show that d is a semi-metric on X. Define f : R2/d ! R by f ([(x, y)]) := x + y. Show that f is an isometry. EXERCISE 1.1.4. Consider the set X of all continuous real-valued functions on [0, 1]. Show that Z 1 d( f , g) := j f (x) − g(x)jdx 0 defines a metric on X. Is this still the case if continuity is weakened to integrabil- ity? EXERCISE 1.1.5. Let (X, dX) and (Y, dY) be two metric spaces. We define q dX×Y((x1, y1), (x2, y2)) := dX(x1, x2) + dY(y1, y2). × Show that (X Y, dX×Y) is a metric space. EXERCISE 1.1.6. As a subspace of R2, the unit circle S1 carries the restricted 2 Euclidean metric from R . We can define another (intrinsic) metric dint by dint(x, y) := the length of the shorter arc between them. 2 p 2 1 Note that dint(x, y) [0, ] for any points x, y S . (a) Show that any circle arc of length less than or equal to p, equipped with the intrinsic metric, is isometric to a straight line segment. (b) The whole circle with the intrinsic metric is not isometric to any subset of R2. 1.1. METRIC SPACES 3 Let (X, d) be a metric space. Set B(x, r) := fy 2 X : d(x, y) < rg, B(x, r) := fy 2 X : d(x, y) ≤ rg. We define the metric topology on X by saying that U ⊂ X is open if and only for any x 2 U there exists e > 0 such that B(x, e) ⊂ U. Let (1.1.1.1) T := fall open subsets in Xg [ fÆ, Xg. The set T satisfies the following (1) U \ V 2 T for any U, V 2 T ; [ 2 T f g ⊂ T (2) i2IUi for any collection Ui i2I ; (3) Æ, X 2 T . In general, we can introduce a topology on any set X.A topology on X is a subset T of 2X satisfying (1) U \ V 2 T for any U, V 2 T ; [ 2 T f g ⊂ T (2) i2IUi for any collection Ui i2I ; (3) Æ, X 2 T . The pair (X, T ) is called the topological space, and any element in T is said to be open; a closed set is a subset of X whose complement in X is open. In particular, we see that any metric space must be a topological space. Given a metric space (X, d). • (X, d) is complete if any Cauchy sequence1 in X has a limit in X. • A diameter of S ⊂ X is defined by (1.1.1.2) diam(S) := sup d(x, y). x,y2S • (X, d) is compact if any sequence in X has a converging subsequence. • ⊂ e f 2 ≤ eg S X is an -net if Se := x X : d(x, S) = infy2X d(x, y) = X. • X is totally bounded if for any e > 0 there exists a finite e-net in X. The following theorem is a basic result. THEOREM 1.1.7. Let (X, d) is a metric space. Then X is compact if and only if X is complete and totally bounded. 1.1.2. Length spaces. Let (X, T ) be a topological space. We say X is Haus- dorff if for any different points x, y 2 X, there exist open sets Ux, Uy ⊂ X such that x 2 Ux, y 2 Uy, and Ux \ Uy = Æ. Clearly that any metric space must be Hausdorff. A path g in X is a continuous2 map g : I ! X. where I ⊂ R is an interval (i.e., connected subset) of R. A length structure on X is a pair (A , L), where A is a collection of paths (called admissible paths) and L : A ! R+ is a map on A (called the length of paths), satisfying 1 f g e 2 A sequence xn n2N in X is said to be Cauchy if for any > 0 there exists an integer n0 N e ≥ such that d(xn, xm) < whenever n, m n0. − 2That is, g 1(U) is open in I for any open set U in X. 4 1. BASIC TOPOLOGY A g ! A gj 2 A (a) ( is closed under restrictions) If : [a, b] X in , then [c,d] for any subinterval [c, d] ⊂ [a, b]; A g ! g (b) ( is closed under concatenations of paths) If 1 : [a, c] X and 2 : ! g g [c, d] X are admissible with 1(c) = 2(c), then the obvious product g g · g ! := 1 2 : [a, d] X is also admissible; (c) (A is closed under reparameterizations) If g : [a, b] ! X is admissible and j : [c, d] ! [a, b] is a homeomorphism, then g ◦ j : [c, d] ! x is also admissible; gj gj gj (d) (Length of paths is additive) L( [a,b]) = L( [a,c]) + L( [c,b]) for any c 2 [a, b] and g : [a, b] ! X in A ; (e) (The length of a piece of a path continuously depends on the piece) If g : [a, b] ! X is a admissible path with L(g) < +¥, then L(g, a, t) := g L( [a,t]) is continuous in t; (f) (L(·) is invariant under reparametrizations) L(g ◦ j) = L(g) for any ad- missible path g and any homeomorphism j as in (c); (g) (Length structures agree with the topology of X) For any x 2 X and open set Ux 3 x, one has inf fL(g) : g(a) = x, g(b) 2 X n U g > 0. g2A x Let (X, T ) is a Hausdorff space with a length structure (A , L). Define (1.1.2.1) d (x, y) := inf fL(g)jg : [a, b] ! X, g(a) = x, g(b) = yg . L g2A ¥ If there is no path between x and y, we set dL(x, y) = + . We say a length struc- A 2 ¥ ture ( , L) is complete if for any x, y X with dL(x, y) < + , there exists a path g 2 A g joining x and y such that dL(x, y) = L( ).
Details
-
File Typepdf
-
Upload Time-
-
Content LanguagesEnglish
-
Upload UserAnonymous/Not logged-in
-
File Pages112 Page
-
File Size-