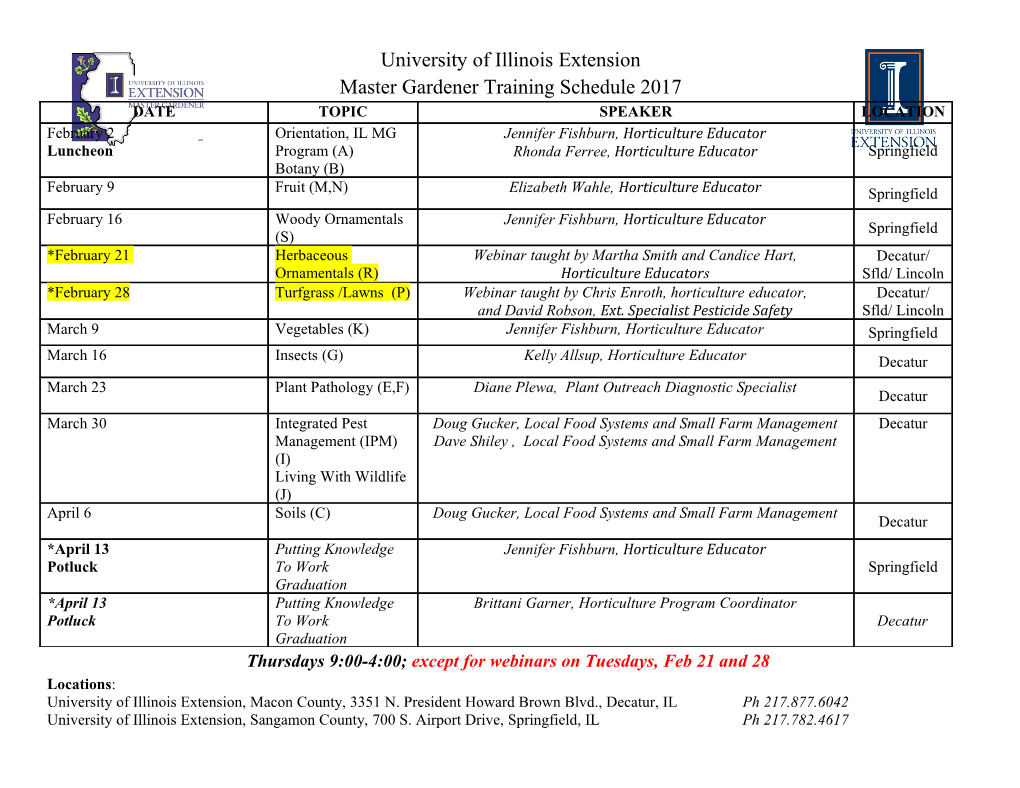
The Space Congress® Proceedings 1984 (21st) New Opportunities In Space Apr 1st, 8:00 AM Magnetic Refrigeration: The Promise and the Problems J. A. Barclay Project Leader, Group P-10, MS-K764, Los Alamos National Laboratory, Los Alamos, NM 87545 Follow this and additional works at: https://commons.erau.edu/space-congress-proceedings Scholarly Commons Citation Barclay, J. A., "Magnetic Refrigeration: The Promise and the Problems" (1984). The Space Congress® Proceedings. 3. https://commons.erau.edu/space-congress-proceedings/proceedings-1984-21st/session-6/3 This Event is brought to you for free and open access by the Conferences at Scholarly Commons. It has been accepted for inclusion in The Space Congress® Proceedings by an authorized administrator of Scholarly Commons. For more information, please contact [email protected]. Magnetic Refrigeration: the Promise and the Problems J. A. Barclay, Project Leader Group P-10, MS-K764 Los Alamos National Laboratory Los Alamos, NM 87545 ABSTRACT tests, but several studies of efficiency as a function of cooling power of gas-based Magnetic refrigeration uses the temperature- refrigerators for cryogens have been and field-dependence of the entropy of some published, including compilations of mass magnetic materials to accomplish cooling. and volume.2-7 The main sources of ineffi­ Because of the intrinsically high efficiency ciency are the room-temperature compressors of the magnetization and demagnetization with their associated aftercoolers and the process and because of the potential for gas expanders. Generally, the compressors excellent heat transfer between solids and and expanders are the least-reliable compo­ fluids, magnetic refrigerators promise to nents too. All of the efficiency studies have higher efficiency than existing gas- agree that ^35% of Carnot efficiency is the cycle refrigerators. Many ground-based and best that is now possible, but then only for space-based applications could benefit very large plants. For smaller machines, significantly from the cost savings implied the fraction of Carnot efficiency can become by higher efficiency. Other attributes of very small, e.g., 1-W refrigerators gener­ these devices are high reliability and low ally operate in the range 2-8% of Carnot. volume and mass per unit cooling power. The One study 5 on liquid hydrogen indicates that development of these refrigerators is under­ no significant advances in liquefaction way at several places around the world, efficiency are probable by the year 2000 including the Los Alamos National Laboratory. unless very large investments are made in The progress to date has been encouraging research and development. Therefore, it is but some problems have been clearly identi­ important to investigate other promising fied. The arguments for high efficiency and technologies to see if they offer higher the problems that will need to be solved to efficiency with less investment. One such achieve this goal are discussed. technology is magnetic refrigeration (MR). MR exploits the temperature and magnetic- field dependence of the magnetic entropy of INTRODUCTION some solid materials to extract heat from a low-temperature source and transfer it to a Important features of refrigerators include higher temperature sink. The temperature efficiency, reliability, lifetime, size, and change associated with the application or mass. The efficient liquefaction of cryo- removal of a magnetic field to an isolated gens, such as liquid hydrogen, is important sample is called the magnetocaloric effect. because the liquefaction cost is a signifi­ This paper presents several reasons why cant fraction of their total cost. The magnetic refrigeration offers significant costs of liquid nitrogen and liquid oxygen promise and identifies some of the problems are also tightly coupled to the cost of that must be overcome before this promise is refrigeration for air separation. At realized. present, all of the refrigeration required to produce cryogens is provided by gas-cycle devices in which gas is compressed in one FUNDAMENTALS OF MAGNETIC REFRIGERATION^ part of the cycle to reject heat from the gas and expanded in another part of the Any refrigeration system requires some mecha­ cycle to cool and eventually liquefy the nism to increase or decrease the entropy of gasJ Lifetime and reliability studies of a working substance. In addition, heat refrigerators are limited to specific design transfer at appropriate times during a cycle 6-10 is necessary. Further analysis of various cycle. From reference 8, the rate of work magnetic refrigerator designs produces a list of a magnetic cycle can be written as of the functions required to execute the thermodynamic cycles. A summary of functions IdT in the basic magnetic cycle over a large • • /TH \ TH)W,(T-T temperature span such as 20 to 77 K is given w = Q ( Jl. i I + —J—L±—— below: \ Tc / magnetization of ferromagnets near the hot temperature to reject heat to a sink such as liquid nitrogen, by circulation of a heat exchange fluid, such as helium J IRR.dT gas; (1) regeneration of the magnetic material to dT cool it to near the cold temperature p (20 K); this regeneration requires heat and the relative efficiency can be written transfer between an external regenera­ as tive material or the magnetic material itself and a moving heat transfer fluid; demagnetization of the ferromagnet near n . (2) the cold temperature to absorb heat from a load, such as hydrogen by circulation of a heat exchange fluid, such as helium where Qc is the reversible cooling power, gas; and Wj is power added externally to move fluid, regeneration of the magnetic material to qj is power introduced through heat conduc­ warm it to near the hot temperature tion from the surroundings, andASiRR is the (77 K); as before, this regeneration rate of irreversible entropy production from requires heat transfer between a regener­ different mechanisms. The irreversibility ator material and a moving heat transfer due to heat transfer across a temperature fluid. difference in the regenerative parts of the The work for the magnetic cycle is provided cycle was retained in the expressions above by a motor working against the difference in and a quantitative expression was evaluated, forces during the magnetization and demagnet­ given as ization. The cycle requires either moving the material or the magnet or some equivlent change. The rate of movement through the WTOTAL = QC | ~ " magnetic cycle is very slow compared to many c gas cycles; ranging from ^0.1 Hz to ^1 Hz. ( TH . THE PROMISE OF MAGNETIC REFRIGERATION dT K" IRR Magnetic refrigeration is an unproven techno­ (3) logy but it does have several attributes which make it worthy of development. The first and perhaps most exciting is the pros­ pect of much higher efficiency than existing gas-cycle refrigerators or liquefiers. In Following the analysis, th,e resultant effi­ general terms, this claim is based upon two ciency expressions were written as arguements. First, the magnetization and demagnetization of a magnetic solid provides •(TH -Tc) an entropy change that is reversible at low (4) frequencies, such as 1 Hz which are the normal operating frequencies of magnetic refrigerators. Secondly, the heat transfer for the temperature range where the heat in magnetic refrigerators is between a solid capacity of the magnetic solid is approxi­ and a gas, which is intrinsically better mately constant. N|U is the number of heat than gas-to-sol id-to-gas, as is required in transfer units in the regenerative-cycle step gas-cycle devices. andATc is the adiabatic temperature change at the cold end of the cycle. The hot and An analysis of the sources of inefficiency cold temperatures are T^ and Tc , respec­ in magnetic cycles^ clearly indicates that tively. When the solid heat capacity is a the biggest fundamental source of irrevers- linear function of temperature, the effi­ ibility in magnetic refrigerators is that ciency expression becomes due to heat transfer across a temperature difference in the regenerative parts of the 6-11 Associated with the above problem is the (5) reduction of the adiabatic temperature change by the thermal addenda of the struc­ ture containing the refrigerent. The problem reduces to generation of clever The analysis in reference 8 shows that designs that can satisfy the structural magnetic refrigerator spanning 4 K to 300 K demands and, at the same time, have can be considered in three ranges on the extremely small thermal mass. basis of regeneration. The range 4-20 K needs little or no regeneration, the range For ferromagnets near their Curie tempera­ 20-150 K needs regeneration where the heat ture, the adiabatic temperature change is capacity of the working solid is approxi­ almost linearly proportional to the field mately linear in T, and the range 150-300 K strength. Therefore, the largest practical needs regeneration where the heat capacity magnetic field must be used to produce the is approximately constant. Projected effi­ largest adiabatic temperature change. For ciencies for several 4-20 K stages are «70% standard solenoids, made from readily avail­ of Carnot. Optimistic estimates of numbers able NbTi superconductor, 9T is an upper for a magnetic refrigerator operating above limit at 4.2 K, but fields above 10 T can be 20 K are N|U «500 andATc «15 K. Substi­ reached if the magnet is cooled to 1.8 K. tution of these values into Eqs. (5) and (4) If Nb3$n is used as the superconductor, for the 20-150 K and 150-300 K range, respec­ magnetic fields of 15 T can be obtained but tively, gives n (20-150 K) = 81% and n at the expense of significant manufacturing (150-300 K) = 98%. When the efficiencies of difficulties. Magnetic refrigerator designs the three stages are combined, the resultant have been made that use racetrack and helm- efficiency is a very impressive 62% of Carnot hoi tz or even more exotic-shaped coils.
Details
-
File Typepdf
-
Upload Time-
-
Content LanguagesEnglish
-
Upload UserAnonymous/Not logged-in
-
File Pages6 Page
-
File Size-