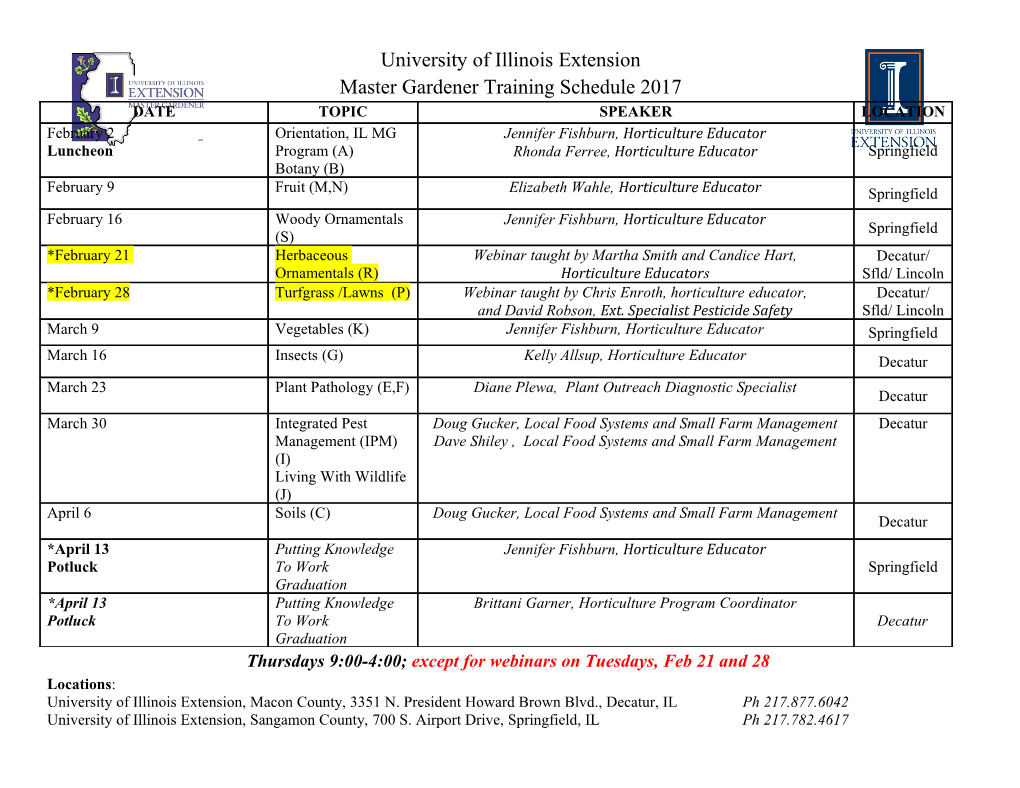
Structure and Function Relationship in Nerve Cells & Membrane Potential Asist. Prof. Aslı AYKAÇ NEU Faculty of Medicine Biophysics Nervous System Cells • Glia – Not specialized for information transfer – Support neurons • Neurons (Nerve Cells) – Receive, process, and transmit information Neurons • Neuron Doctrine – The neuron is the functional unit of the nervous system • Specialized cell type – have very diverse in structure and function Neuron: Structure/Function • designed to receive, process, and transmit information – Dendrites: receive information from other neurons – Soma: “cell body,” contains necessary cellular machinery, signals integrated prior to axon hillock – Axon: transmits information to other cells (neurons, muscles, glands) • Information travels in one direction – Dendrite → soma → axon How do neurons work? • Function – Receive, process, and transmit information – Conduct unidirectional information transfer • Signals – Chemical – Electrical Membrane Potential Because of motion of positive and negative ions in the body, electric current generated by living tissues. What are these electrical signals? • receptor potentials • synaptic potentials • action potentials Why are these electrical signals important? These signals are all produced by temporary changes in the current flow into and out of cell that drives the electrical potential across the cell membrane away from its resting value. The resting membrane potential The electrical membrane potential across the membrane in the absence of signaling activity. Two type of ions channels in the membrane – Non gated channels: always open, important in maintaining the resting membrane potential – Gated channels: open/close (when the membrane is at rest, most gated channels are closed) Learning Objectives • How transient electrical signals are generated • Discuss how the nongated ion channels establish the resting potential. • How the flux channels of ions through gated channels generates the action potential. • Illustrate how the channels, along with other component important for nerve cell signaling • Represented by an electrical equivalent circuit. Membrane potential results from the seperation of charge across the cell membrane • A nerve cell at rest has an excess of positive charges on the outside of the membrane and an excess of negative charges on inside. • This charge separation brings about electrical potential difference across the membrane. • The potential difference is called the resting membrane potential. When the cell is at rest, the resting membrane potential applies only to the potential across the membrane. • The more general term membrane potential refers to the electrical potential difference across the membrane at any moment in time. • By convention, the potential outside the cell is randomly defined as zero, so membrane potential (Vm) is defined as Vm = Vin-Vout The resting membrane potential is determined by different types of non-gated ions channels Cell Membrane Cytoplasm - + Extracellular - + + + [Na ] = 15 mM - + [Na ] = 145 mM + [K+] = 150 mM - + [K ] = 5 mM [Cl-] = 9 mM - + [Cl-] = 125 mM - + Nongated channels in glia cells are selective only for potassium. • The membrane of glia cells have nongated channels. • Most of nongated channels are selectively permeable to K+ ion. • In most of glia cells, membrane potential is about -75mV. Nernst Equation Calculates the equilibrium potential for each ion E= -2,3 R = gas constant, T = temperature in degrees Kelvin, F = Faraday constant, z = charge of the ion Nongated channels in nerve cells are selective for several ion species Nerve cells at rest, unlike glia cells, are permeable to Na+ , K+ and Cl- ions. The passive fluxes of Na+ and K+ through nongated channels are balanced by active pumping of Na + /K + ions • For the cell to have resting membrane potential, the charge separation across the membrane must be constant. • Therefore, for the cell to achieve a resting state, the movement of K + out of the cell must balance the movement of Na + into the cell. The charge separation across the membrane must be constant. The balance provided by active pumping of Na + /K + ions. The action potential is generated by the opening of voltage-gated channels selective for Na+ and K + • In nerve cell at rest • Na+ influx through nongated channels is balanced by a steady efflux • so the membrane potential is constant. • A transient depolarizing potential • causes some voltage-gated Na+ channels to open. • The resultant increase in membrane Na+ permeability allows Na+ influx to outstrip the K+ efflux. The action potential is generated by the opening of V-gated channels selective for Na+ and K + A net influx of position charge flows through the membrane, and positive charges accumulate inside the cell, causing further depolarization. Resting Membrane Potential • Goldman-Hodgkin-Katz Equation – Takes into account all ionic species and calculates the membrane potential Permeability: PK: PNa: PCl = 1 : 0.04 : 0.45 Cl- typically not pumped, so at equilibrium K+ dominates because the greatest conductance Resting membrane potential usually very negative about -70 mV E.g. • In nerve cell: pNa+ / pK + = 0.06 rest pot = -70mV • In muscle cell: pNa + / pK + = 0.01 rest pot = -90mV Electrical Signals-1 • Deviation in the membrane potential of the cell – Depolarization • Reduction of charge separation across membrane • Less negative membrane potential – Hyperpolarization • Increase in charge separation across membrane • More negative membrane potential • Cause: Ion channels open/close – Large change in permeability of ions relative to each other – Changes in net separation of charge across cell membrane Electrical Signals-2 • Spread according to different mechanisms – Electrotonic conduction • Dendrites – Action Potential • Axons Electrotonic Conduction-1 The passive spread of voltage changes along the neuron is called “electrotonic” conduction. Electrotonic conduction is important in the propagation of action potential. Electrotonic Conduction-2 • Na+ channels opened – Na + flows into cell – Membrane potential shifts toward Na + equilibrium potential (positive) Na+ – Depolarization Information Processing • A single neuron receives inputs from many other neurons – Input locations • Dendrites – principle site • Soma – low occurance Transmitting Information • Signal inputs do not always elicit an output signal – Change in membrane potential must exceed the threshold potential for an action potential to be produced – Mylenated axons • Axon hillock = trigger zone for axon potential – Unmyelenated axons • Action potentials can be triggered anywhere along axon Axon hillock Action Potential Action potential is seen in axon hillock. normally propagates from the axon hillock along the axon. works ‘All-or-none’ principle. * axon hillock: The initial segment of the axon. • Action potential normally propagates from the axon hillock along the axon. Time Propagation Direction Propagation of the Action Potential If excitation is initiated artificially somewhere along the axon, propagation then takes place in both directions from the stimulus site. stimulation Propagation Direction Propagation of The Action Potential Steps An action potential is generated in any region. .As a response, voltage-gated Na+ channels are opened. .This local depolarization then spreads electrotonically along the axon. .The voltage-sensitive channels in the new (next) location will go through the same sequence previously described regenerating the action potential. Subsequent portions of the axons are depolarized in the same manner. .Strong depolarization in one area results in depolarization above the threshold in neighboring areas. Modeling Neurons The current flow in a neuron can be modeled by an electrical equivalent circuit. • Neurons are electrically active • Model as an electrical circuit – Battery :Current (I) generator, + + Na /K pump + + – Resistor: Leak channels Battery i Resistor + + - - + for Na , K , Cl ions. - + - + - + – Capacitor: Lipid bilayer Capacitor – Generators: Ions currents (inward or outward) from leak channels. Neuron modeled as an electrical circuit If we consider a neuronal membrane at the rest; Extracellular side I ++++ K+ INa+ RNa+ R R Cm Cl- K+ ------ + + - - + ENa+ - ECl- EK+ + + pump Cytoplasmic side Na /K Ionic Gradients as Batteries • Concentration of ions differ between inside the neuron and outside the neuron • Ion channels permeate the membrane – Selective for passage of certain ions – Vary in their permeability – Always open to some degree = “leaky” • Net Result: each ionic gradient acts as a battery – Battery • Source of electric potential • An electromotive force generated by differences in chemical potentials Ion Channels as Resistors • Resistor – Device that impedes current flow • Generates resistance (R) • Ion channels vary in their permeability – “Leaky” • Always permeable to some degree – Permeability is proportional to conductivity • Leak channels – conductance relationship g • Conductance (g) = 1/R Membranes as Capacitors-1 • Capacitor – A simple capasitor consist of two metal plates separated by an insulated material. – Each plate is a conducter, at constant potential. – Potential difference between the plates is V. Membranes as Capacitors-2 • Plasma Membrane – Lipid bilayer acts like an insulator separating two conducting media: 1. The external medium of the axon 2. The internal medium of the axon . Which properties of the membrane affect the speed of action potential propagation? Speed of electrotonic
Details
-
File Typepdf
-
Upload Time-
-
Content LanguagesEnglish
-
Upload UserAnonymous/Not logged-in
-
File Pages56 Page
-
File Size-