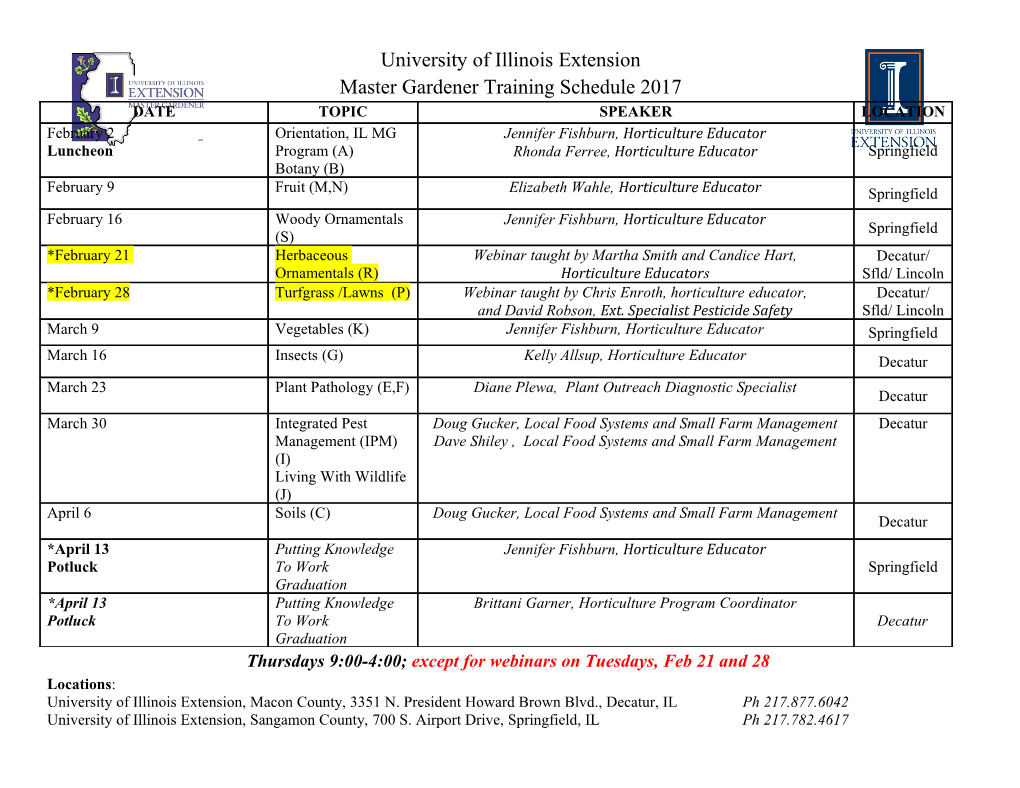
bioRxiv preprint doi: https://doi.org/10.1101/766170; this version posted August 14, 2020. The copyright holder for this preprint (which was not certified by peer review) is the author/funder, who has granted bioRxiv a license to display the preprint in perpetuity. It is made available under aCC-BY-NC 4.0 International license. Visual properties of human retinal ganglion cells Katja Reinhard1,2,3* & Thomas A. Münch1,4* 1 Retinal Circuits and Optogenetics, Centre for Integrative Neuroscience and Bernstein Center for Computational Neuroscience, University of Tübingen, Otfried-Müller-Str. 25, 72076 Tübingen, Germany; 2 Neuroscience Graduate School, University of Tübingen, Österbergstraße 3, 72074 Tübingen Germany; 3 Current address: Neuro- electronics Research Flanders, Kapeldreef 75, 3001 Leuven, Belgium; 4 Institute for Ophthalmic Research, University of Tübingen, Elfriede-Aulhorn-Straße 7, 72076 Tübingen, Germany * Corresponding authors Katja Reinhard: [email protected] and Thomas A. Münch: [email protected] The retinal output is the sole source of visual information for the brain. Studies in non-primate mammals estimate that this information is carried by several dozens of retinal ganglion cell types, each informing the brain about different aspects of a visual scene. Even though morphological studies of primate retina suggest a similar diversity of ganglion cell types, research has focused on the function of only a few cell types. In human retina, recordings from individual cells are anecdotal or focus on a small subset of identified types. Here, we present the first systematic ex-vivo recording of light responses from 342 ganglion cells in human retinas obtained from donors. We find a great variety in the human retinal output in terms of preferences for positive or negative contrast, spatio-temporal frequency encoding, contrast sensitivity, and speed tuning. Some human ganglion cells showed similar response behavior as known cell types in other primates, while we also recorded light responses that have not been described previously. This first extensive description of the human retinal output should facilitate interpretation of primate data and comparison to other mammalian species, and it lays the basis for the use of ex-vivo human retina for in-vitro analysis of novel treatment approaches. Introduction Vision starts in the retina, a highly structured part of the central nervous system. The retina performs important signal processing: the incoming images are captured by the photoreceptors, analyzed and split into parallel information streams by retinal circuits, and sent along the optic nerve to higher visual brain centers. Each of the parallel information streams is embodied by a type of ganglion cell and informs the brain about a particular aspect of the visual scene [1]. The non-primate mammalian retina contains over 40 of these different information streams, which can be distinguished based on both functional and morphological criteria [2–7]. One striking aspect of retinal architecture is that each ganglion cell type tiles the retina so that each feature can be extracted at each location in the visual field. Nevertheless, regional specializations do exist, for example the fovea of the primate retina, a region of very high visual acuity. The foveal region consists almost exclusively of four retinal ganglion cell types, the ON and OFF parasol cells, and the ON and OFF midget cells [8–10], which account for 50-70% of all ganglion cells in the primate retina [11]. Functional studies using human and non-human primates have often focused on these four most abundant retinal ganglion cell types [12–19]. Morphological studies of the complete primate retina, on the other hand, describe a similar variety in ganglion cell types as found in the non-primate retina with at least 17 morphologically identified types [11,20–23]. However, functional studies of these non-foveal ganglion cell types in non-human primates have been limited to a set of 7 types [14,24–29] and only midget and parasol cells have been recorded in human retina [18,19]. Additional physiological assessment of the human retina on the level of individual cells is anecdotal [30,31]. In this study, we present a survey of ganglion cell function in the non-foveal human retina. We performed multi-electrode array (MEA) recordings on ex-vivo retinas obtained from enucleation patients 1 bioRxiv preprint doi: https://doi.org/10.1101/766170; this version posted August 14, 2020. The copyright holder for this preprint (which was not certified by peer review) is the author/funder, who has granted bioRxiv a license to display the preprint in perpetuity. It is made available under aCC-BY-NC 4.0 International license. and recorded light-driven activity from dozens of human ganglion cells in parallel. MEAs have been successfully used in previous studies to characterize the retinal output in various animal models [4,12,32–37]. Our data represents the first systematic and non-selective recording and characterization of light responses from a large population of ganglion cells in human retina. In addition to providing an overview of the spectrum of light responses in the human retina, we compare the representation of the spatio-temporal stimulus space by human ganglion cells with published data from non-human primate retina and results from psychophysical studies. Results Recording light responses from donated human retinas To record light responses from human retinal ganglion cells, we obtained human retinas from patients who had to undergo enucleation of one eye due to a uveal tumor. Retinal pieces (~ 3 x 3 mm²) were placed ganglion cell-side down onto multi-electrode arrays and responses to a set of light stimuli were recorded at photopic light intensities. Individual stimuli (gray-scale images) spanned at most 3 log units of brightness. Spikes were assigned to individual units (presumably retinal ganglion cells) during an offline, semi-manual spike sorting process based on principal component analysis of spike waveforms. Only clearly sortable units were considered for analysis (see Method section for details). In total, we obtained the spiking activity of 342 light-responsive single units in 15 retinal pieces obtained from 10 human retinas (Table 1). Table 1: Human retinas used for this study. Donor Surgery conditions Retina Experiment notes Retinal part Any Ischemia Dark # Analyzed ID Sex Age Notes Eye (ventr./dors./ Preparation light (min) lens pieces temp./nasal) resp.? 1 m 72 diabetic 7 - right vt easy 1 yes 2 f 49 radiation 7 - right dn - - 3 f 72 diabetic 7 - left dn rolling 1 yes 4 f 69 detachment 17 - left n easy 1 yes 5 m 53 7 - left t sticky 1 yes *6 m 75 sinus tumor 18 - - - 7 m 89 25 - right dn sticky - yes 8 f 42 20 - left vt sticky - - 9 f 83 10 - right t sticky 1 yes 10 f 49 10 - left t sticky 3 yes 11 f 60 7 yes left vn sticky 3 yes 12 f 74 macular edema 7 yes left dt easy 2 yes 13 m 74 7 yes left v very sticky - yes 14 f 79 radiation 10y ago 8 - left n easy 1 yes 15 m 67 detachment 10 - left n easy 1 yes 15 ex-vivo human retinas were obtained. The table contains information about the donor (sex, age, known medical history), surgery conditions (the ischemia duration, i.e. time without oxygen and nutrient supply, and whether a dark lens was put on the donor’s eye during surgery), and the retina (left/right eye, part of the retina without tumor). During preparation, the retina would sometimes roll up immediately after vitrectomy (rolling) or the vitreous was sticking strongly to the retina (sticky). The last two columns indicate how many retinal pieces were used per retina for the final analysis and whether any light responses were detected in our recordings. Light gray rows: retinas with few light responses, not used for analysis. Dark gray rows: no detectable light responses. Bold: potential reasons for low quality. Ventr./v = ventral, dors./d = dorsal, temp./t = temporal, n = nasal, m = male, f = female, radiation = radiation of the tumor-bearing eye, detachment = partial retinal detachment prior to surgery, resp. = responses. *retina prepared by another group during a different study. 2 bioRxiv preprint doi: https://doi.org/10.1101/766170; this version posted August 14, 2020. The copyright holder for this preprint (which was not certified by peer review) is the author/funder, who has granted bioRxiv a license to display the preprint in perpetuity. It is made available under aCC-BY-NC 4.0 International license. Figure 1: Population response of human retinal ganglion cells. Four different types of stimuli were used (A-D). A) Drifting gratings with 4 temporal frequencies and 6 spatial frequencies. B) Full-field “chirp” frequency ramp from 0.5 to 8 Hz and full-field “chirp” contrast ramp. C) Bar moving with 6 different speeds. D) Full-field flash contrast steps consisting of two positive and two negative contrast steps. (E) Response strength (amplitude of the Fourier Transform (FT) normalized to maximal response) to 24 drifting sinusoidal gratings with different spatial and temporal frequencies, averaged across N = 293 cells. (F) Distribution of preferred spatial (left) and temporal (right) frequencies in response to drifting gratings, N = 293 cells. (G) Normalized response strength (FTresponse/FTstimulus) to a full-field frequency ramp (“chirp”), in different frequency bands (Box-whisker-plots: mean, quartiles, maximum and minimum; N = 141 cells). (H) Distribution of the median preferred speed measured with a single moving bar, N = 37 cells. (I) Proportion of ganglion cells responding to positive full-field contrast steps (ON), negative contrast steps (OFF) or both (ON-OFF), N = 121 cells. Response properties across the population of ganglion cells We aimed at characterizing the diversity of the output of the human retina with different visual stimuli (Fig.
Details
-
File Typepdf
-
Upload Time-
-
Content LanguagesEnglish
-
Upload UserAnonymous/Not logged-in
-
File Pages19 Page
-
File Size-