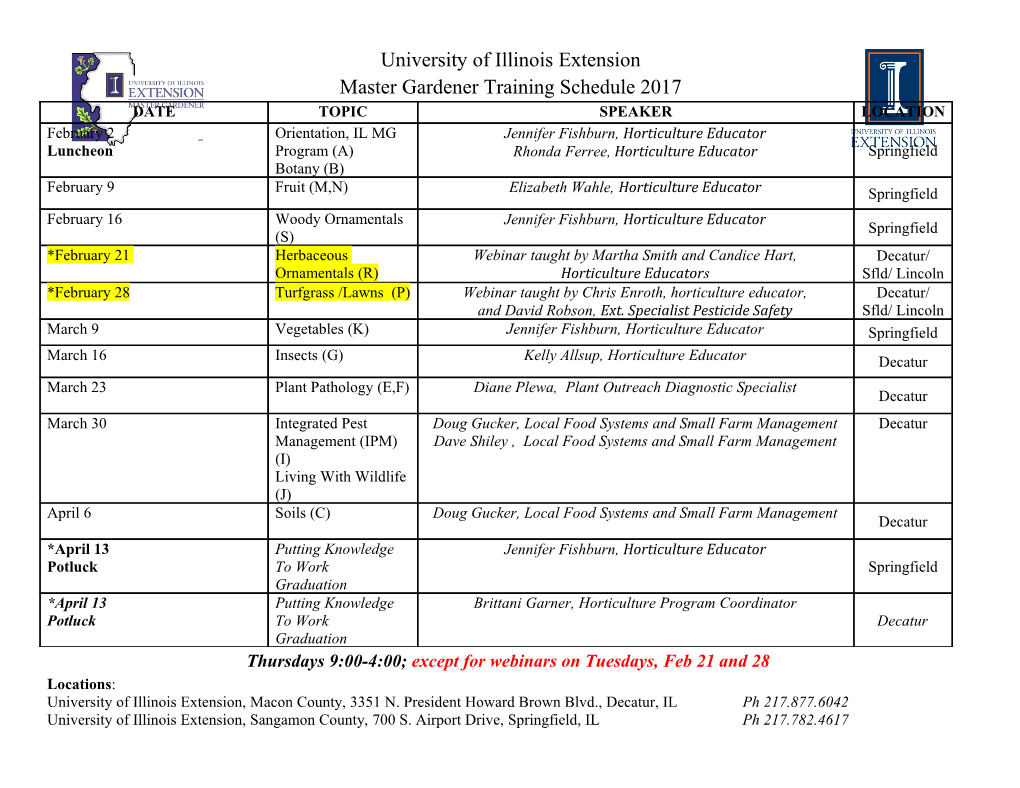
Electrons that tunnel through insulating barriers and hop on and off minuscule dots are advanced technology at the heart of future transistor generations Quantum transistors: toward nanoelectronics LINDA GEPPERT “THE SMALLER WE ARE, the better we perform.” Quantum cellular automata are a fourth type of Senior Editor That is the siren song of quantum transistors, in device. Automata are cells that contain four quan- which electrons skip on and off quantum dots or tum dots arranged in a square. An extra electron tunnel through barriers thought impenetrable in resides on each dot of one diagonal or the other, the world of classical physics. determining if the cell stores a logic 1 or a 0. The Nothing stands between these devices and ever- cells perform the necessary logic functions by inter- increasing density and performance. Manufacturing acting with neighboring cells. The dots can be metal processes keep on shrinking their feature sizes, even but arrangements of molecules are also possible. down to atomic-scale dimensions, and switching Though quantum transistors are a novelty today, frequencies approaching a terahertz are foresee- they will be needed once the classical field-effect able because only a handful of electrons is needed transistor (FET) can be made no smaller—an event to operate the devices. even now on engineers’ radar screens. “Already in So great is the allure of quantum devices that sev- research labs around the world the last generation eral types are under development. One variant is of bulk CMOS is being explored,” wrote Hon-Sum the double–electron-layer tunneling transistor Philip Wong in the April 1999 Proceedings of the IEEE. (Deltt) built by researchers at Sandia National What will eventually stop CMOS technology Laboratories, in Albuquerque, N.M. Another avenue in its tracks is not the inability to shrink its physi- is to boost the performance of conventional tran- cal size further, but the dire effect of quantum phe- sistors by teaming them with resonant tunnel nomena on the ever-tinier transistor’s operation. In diodes, quantum devices similar to the Deltts. So nanoscale FETs, tunneling through ultrathin oxides far, most resonant tunneling devices (both Deltts and extremely narrow channels leaks an unaccept- and diodes) have utilized indium phosphide or gal- able amount of current. And the minuscule number lium arsenide processes. But engineers are busy of dopant atoms in the channel varies enough from building silicon-based devices as well. one transistor to the next to wreak unavoidable Another sort of quantum device shows great havoc with operating margins [see “The incredible promise for nonvolatile memory. Called the single- shrinking transistor,” Yuan Taur, IEEE Spectrum, July electron transistor, or sometimes the quantum dot 1999, pp. 25–29]. transistor, it is under development by research groups “Currently we are hoping that we can get down worldwide. A single-electron memory cell—the to 20- or 30-nm channel lengths. But below that nanocrystal device pioneered by Sandip Tiwari, now it looks very difficult to continue with CMOS,” at Cornell University, Ithaca, N.Y.—is of silicon, Wong’s co-author, David J. Frank, told Spectrum operates at room temperature, and should prove recently. Frank is a research staff member at IBM to have faster read and write times than conventional Corp.’s Thomas J. Watson Research Center, in nonvolatile memories. Yorktown Heights, N.Y. 46 0018-9235/00/$10.00©2000 IEEE IEEE SPECTRUM SEPTEMBER 2000 ELECTRONS IN A WELL on the other side of the barrier is equal in energy and momentum Perhaps the most striking quantum effect in transistor-like to the state the electron originally occupied. devices is tunneling. The term alludes to a particle plunging In general, when no voltage is applied to the device, there are through a barrier that would be impenetrable in the classical world. no matching states in the two wells, and the device is off. But when The mechanism is basic to the Deltt being developed at Sandia. the energy level of the electrons in one well is shifted appropri- Though Deltt developers are still in the early stages of explo- ately, the energy states line up opposite each other and tunneling ration, they are pinning their hopes for high speed on performance occurs—a condition known as resonance. Applying voltage to a already obtained with devices that are similar except in having control gate or biasing one of the wells relative to the other will two terminals rather than three. Called resonant tunnel diodes, shift the energies. In practice, both voltages are applied in oper- they have been shown to oscillate at frequencies up to 700 GHz. ating the Deltt. Further, Deltts lend themselves to volume manufacturing. They are built with a planar process, using conventional semiconduc- THE BIG DIFFERENCE tor deposition. As deposition can be controlled to within a few In one way, the Deltt’s operation is like the switching of an ordi- tenths of a nanometer, it is more than adequate for all structures nary transistor: at a certain source-drain bias voltage, the device with dimensions critical to Deltt operation. can be switched on or off by applying a voltage to the gate. But Structurally, the Deltt positions an insulating barrier between there is one important difference. Raise the gate or drain voltage two two-dimensional wells. In operation, electrons quantum- on a conventional transistor, and current increases. But in a Deltt, mechanically tunnel from one well to the other through the barrier raising the voltage beyond the point of resonance shuts off the [Fig. 1]. Device speed benefits from the tunneling process, which current [Fig. 2]. Put another way, the differential resistance— is much faster than the drift of electrons, let alone of slower holes, the change in voltage with respect to a change in current—can across a channel. Sandia researchers label the two wells as source become negative. This feature allows complementary circuits to and drain, to correspond with conventional transistors. be built with only one type of transistor, rather than the n- and A well is formed by surrounding one region holding a bevy p-type transistors required for CMOS circuits. “It’s a unipolar of free-electron energy states with another region having a dearth device—the only carriers are electrons. But because the transcon- of them. An example might be an insulator or a wide-bandgap ductance can take either sign, depending on the control gate volt- semiconductor, say, surrounding a metal or narrow-bandgap semi- age, I can make complementary circuits,” explained Jerry Simmons, conductor. The well is deemed two-dimensional when it is so nar- who developed the Deltt. He is Sandia’s manager of the semi- row in one dimension that electron motion is restricted to the conductor material and device sciences department. plane of the well. “That is the exciting thing about these devices,” he said, “They The dimensions of the well and the height of the barrier (defined are multifunctional, which means that you can perform the same as the energy an electron needs to pass over it) determine the dis- circuit functions with fewer devices.” As an example, the Sandia crete energy states allowed to the electrons in the well. An elec- researchers built a static RAM cell using two Deltts in series tron can tunnel through the barrier only if its energy and its momen- [Fig. 3]. A conventional CMOS static RAM cell requires n- and tum in the plane of the well are both conserved. In other words, p-type transistors, complicating fabrication. an electron can tunnel only if the energy state of the quantum well Sandia’s Deltt still has a way to go. For one thing, the milli- Top quantum well contact (source) Top control gate Top depletion gate Top quantum well Barrier Tunneling Bottom quantum well Bottom quantum well contact (drain) Back depletion gate Back control gate (optional) [1] The double–layer tunneling transistor, or Deltt, is a planar minals contact the top and bottom well, respectively. A top device consisting of two 15-nm-thick quantum wells separated depletion gate rids the top quantum well of electrons in the by a 12.5-nm-thick barrier. Electron tunneling between the region next to the drain [yellow shaded area] to ensure that wells is controlled by the voltage bias between them and volt- the drain contacts only the bottom quantum well. A back age applied to the top control gate. The source and drain ter- depletion gate does the same for the source terminal. 47 volt levels at which it operates not only are hard to integrate with As a remedy, Simmons and his team have added a third well the 1-V level of today’s electronics but also mean susceptibility to to the device. In the new configuration, electrons tunnel from the noise. “The final thing,” said Simmons, “is that the speed is limited first to the second well, then continue through a second tunnel by the Deltt’s RC time constant.” It is easy to shrink the resistance barrier to the third, very wide well. The large voltage drop across by using a very thin barrier, but then the two wells are so close the wide third well allows the electrons to pick up speed. that the capacitance is large. “We have recently demonstrated such a structure working at voltages on the order of a volt, compatible with existing elec- tronics,” said Simmons. “And the source-drain capacitance is much smaller because the starting and ending layers of electrons are very 3 0.4 far apart compared to the two wells.” Simmons has also managed to raise the operating temperature.
Details
-
File Typepdf
-
Upload Time-
-
Content LanguagesEnglish
-
Upload UserAnonymous/Not logged-in
-
File Pages6 Page
-
File Size-