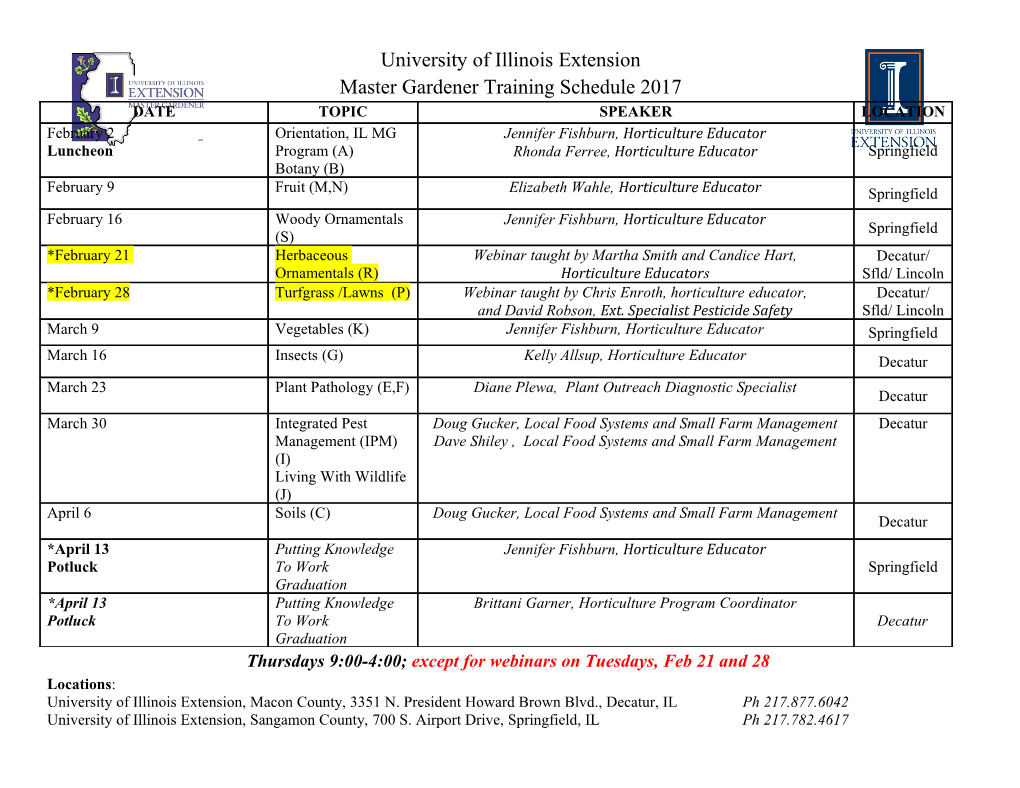
Organosilicon Compounds DR. ABHINAV KUMAR DEPARTMENT OF CHEMISTRY UNIVERSITY OF LUCKNOW Properties of Silicon • The position of silicon in the upper centre of the Periodic Table classifies it as the gentlest amongst the metals. • It possesses some metallic features of the heavier elements of its group, with only very small tendency to form stable divalent derivatives and conform with the inert pair effect. • It dissolves in aqueous alkali giving orthosilicates and also generates hydrogen from hydrofluoric acid, thereby indicating distinct amphoteric behaviour. • Much of the chemistry of silicon has therefore been considered in the light of elements only mildly metallic in character and the study of organosilicon compounds was the prerogative of organic chemists until Rochow introduced the inorganic silicate skeleton. • Silicones were developed to supersede organic polymers, and inorganic chemists played an increasing role in developing the chemistry of substituted organosilicon compounds, e.g. R3SiX and R2SiX2 where X ≠ C. The gamut of these compounds is now being used extensively by organic chemists as synthetic intermediates. • Substitution at silicon is much easier than that at carbon. Attack by protic bases and by organic nucleophiles makes chlorosilanes excellent synthetic intermediates. • This stems from the high rate of bimolecular nucleophilic substitution which typifies a second period element, and takes place with much poorer leaving groups than substitution at carbon. Thus the Si—F, Si—OR, Si—C and Si—H bonds can all be cleaved by the appropriate nucleophile. • The Si—F bond is one of the strongest encountered with the Si—O and Si—Cl bonds significantly stronger than the rest. • The extensive use of silicon compounds as synthetic intermediates in inorganic chemistry is based on the facile cleavage of Si—C, Si—N and Si—S bonds by chlorides of both main group and transition metals. Organic compounds silylated at carbon or oxygen can be readily regenerated using fluoride ion. • Multiple bonds to silicon involving (p-p)π bonding have long been assumed to be weak, partly because of size disparity, but also because the Si—X bond is much longer than the C—X one, thereby decreasing π-overlap. • The weakness of the silicon-carbon double bond precludes competitive elimination in methylchlorosilanes by methoxide, in sharp contrast to its reaction with t-butyl chloride Preparation of Chlorosilanes • Before the manufacture of methylchlorosilanes in the 1940s by the Rochow process, silicon tetrachloride was the main starting material. • It can be prepared directly from the elements, or from silica, coke and chlorine, and though this is a high temperature synthesis, ingredients are cheap. • Ease of nucleophilic attack renders stepwise substitution of silicon tetrachloride by the Grignard reagent or organolithium compounds a facile process. • In 1904, all ethyl- and phenyl-chlorosilanes were prepared from silicon tetrachloride and the Grignard reagent. • However the reactivity of the chloride decreases with progressive substitution, so a particular chloride cannot normally be produced as the only product. • Adding 2.25 mol EtMgCl to one of SiCl4 produces mainly EtSiCl3 and Et3SiCl. • Diethyldichlorosilane represents only some 12% of the chlorosilanes produced. • The competitive alkylation of an equimolar mixture of trimethyl-and triethyl-chlorosilanes gives a 2:1 mixture of Me3SiEt and Et4Si, n n while Pr MgBr gives Me3SiPr only. • Stepwise substitution is easier with the more hindered Grignard and organolithium reagents. • Diphenyldichlorosilane and /-butyltrichlorosilane result as major products in the appropriate 2:1 and 1:1 reactions with SiCl4. • t-butyllithium and dimethyldichlorosilane give t- butyldimethylchlorosilane in good yield. Like many of the mono-t- butylsilanes, this is a crystalline solid, and has been extensively used in organic synthesis to protect alcohols. • Methylchlorosilanes cannot be conveniently prepared by the Grignard reagent/SiCl4 method. • In forming the least hindered of the organochlorosilanes, randomization is greatest and with the four chloro components boiling within 13 °C of each other, separation of these and of the complexing diethyl ether is difficult. Rochow Process • It involves the direct synthesis of chlorosilanes from an alkyl or aryl chloride and silicon in the presence of a copper catalyst, is now used commercially to produce them on a large scale. • The conditions are more vigorous than the usual reaction of an organic halide with a metal, for example magnesium or zinc, but with silicon being so mildly metallic, most silicon- carbon bonds are stable to the normal conditions of hydrolysis. • Care has to be taken separating the methylchlorosilanes, since the properties of silicone polymers produced from these halides are critically dependent upon the composition of the chloride mixture being hydrolysed, so an extensive fractionating system is employed industrially to separate these methylchlorosilanes and silicon tetrachloride • The array of more than 40 by-products tends to support a mechanism in Rochow Process involving organic radical intermediates, but more recent results indicate a chemisorption process in which the dissociative adsorption of the organic halide on the silicon-copper centre appears to be of decisive importance. • Suffice it to say here that many of the 40 products resulting from the production of dimethyldichlorosilane are formed in very small yields, since the major product is normally between 70 and 90% of the total. • Nevertheless with dimethyldichlorosilane now a bulk chemical, some by-products result in significant quantities, and include hydrosilanes (MeHSiCl2, Me2SiHCl, and HSiCl3), methylchlorodisilanes and bis(methylchlorosilyl)methanes as well as a variety of chloroalkanes. Yields of particular by-products can be increased by changing the catalyst and reaction conditions. • The preparation of ethylchlorosilanes by the same method is easier but more random, with significant yields of ethyltrichloro- and ethyldichloro-silane resulting if the ratio of chloroethane to silicon is two. • Trichlorosilane HSiCl3 is a by-product of the Rochow process and is readily converted to triorganosilanes using the Grignard reagent of relatively unhindered halides. Isopropylmagnesium chloride only substitutes two chlorine atoms and the more reactive alkyllithium is needed to replace the third. • Halosilanes can then be prepared directly using the free halogen (not fluorine). The reaction takes place conveniently in carbon tetrachloride at 0 °C, though it is faster in polar solvents. It occurs with retention of configuration at silicon, possibly through flank attack at the Si—H bond, so is relatively unaffected by steric hindrance, in contrast to nucleophilic attack at silicon. • This method usually gives only products of monosubstitution, thereby providing a useful synthetic route to mixed halohydrides and to the more hindered trialkylsilanes and their halides. Thus tri-t-butylchlorosilane can be readily prepared from di-t-butylsilane by stepwise chlorination and alkylation with ButLi, whereas a poor yield results from di-t-butyldichlorosilane and ButLi • Silanes can be polyhalogenated using hydrogen halides, while triorganohalosilanes result in good yield from the reaction of R3SiH with alkyl or acyl halides. • Fluorosilanes can be prepared conveniently at room temperature using a palladium-carbon catalyst, or by heating the hydride with antimony trifluoride Preparation of Si-C bond • The silicon-carbon bond can be prepared by a wide variety of methods and several have been extensively studied. • The most important laboratory method, comprehensively surveyed, involves the reaction of an organometallic carbon nucleophile such as a Grignard reagent or an organolithium compound with chloro- or alkoxy-silanes. • The facile reaction of the Grignard reagent with chlorosilanes has the advantage of leaving reactive functional groups bonded to carbon unaffected. • However with perchlorosilylalkanes, the carbon- chlorine bond is both reduced and alkylated. • Halogen exchange between the silane and Grignard reagent has also been observed. Some more reactions Alkylsilanes • Tetraalkyl silanes are remarkably stable compounds. The silicon-carbon bond is strong and almost non-polar. It is therefore only broken under the most vigorous conditions, unless assisted by an alkyl group possessing an activating substituent suitably placed. Tetramethylsilane can be heated to 700 °C before rapid homolysis occurs, and both tetraphenylsilane and phenyltri(p-diphenyl) silane can be distilled open to the air at 430 °C and 570 °C respectively. Tetraethyl and tetrapropyl-silanes decompose at temperatures some 100 °C below that necessary for tetramethylsilane. • The thermal decomposition of tetramethylsilane has been extensively studied, and the 45 components trapped below 110 °C/2 mmHg alone reflect its complexity without considering all those of higher boiling point. • Both linear and cyclic compounds result with bis(trimethylsilyl)methane the main one, and most contain alternating carbon and silicon atoms. The primary dissociation involves homolysis of the carbon-silicon bond. The methyl radical then extracts hydrogen to provide the precursor for bis(trimethylsilyl)methane and the cyclic products. No disilanes were observed, in part because the silicon-silicon bond is weaker than the carbon-carbon bond Si-C bond cleavage • Strong bases are needed to cleave alkyl groups from silicon, but the reaction
Details
-
File Typepdf
-
Upload Time-
-
Content LanguagesEnglish
-
Upload UserAnonymous/Not logged-in
-
File Pages34 Page
-
File Size-