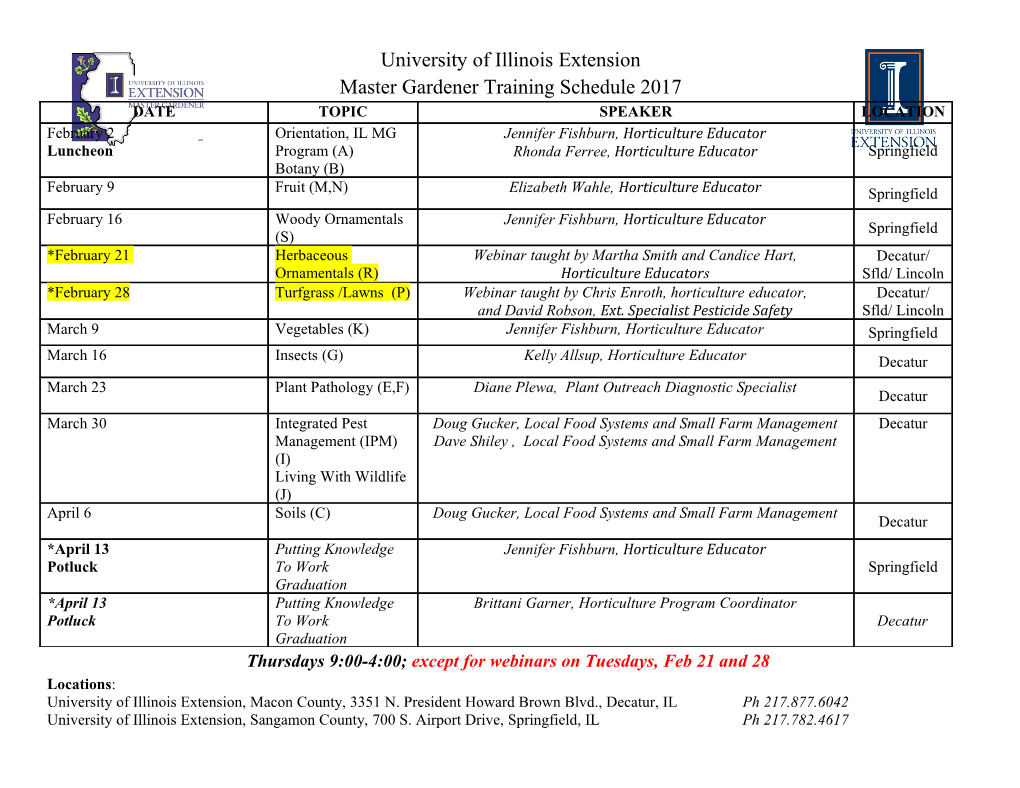
International Journal of Molecular Sciences Review Derivatives of Natural Chlorophylls as Agents for Antimicrobial Photodynamic Therapy Nikita Suvorov 1,*, Viktor Pogorilyy 1 , Ekaterina Diachkova 2,* , Yuri Vasil’ev 3, Andrey Mironov 1 and Mikhail Grin 1 1 Department of Chemistry and Technology of Biologically Active Compounds, Medicinal and Organic Chemistry, Institute of Fine Chemical Technology, MIREA-Russian Technological University, 119571 Moscow, Russia; [email protected] (V.P.); [email protected] (A.M.); [email protected] (M.G.) 2 Department of Oral Surgery of Bororovsky Institute of Dentistry, II.M. Sechenov First Moscow State Medical University (Sechenov University), Trubetskaya St. bldg. 8n2, 119435 Moscow, Russia 3 Department of Operative Surgery and Topographic Anatomy, I.M. Sechenov First Moscow State Medical University (Sechenov University), Trubetskaya St. bldg. 8n2, 119435 Moscow, Russia; [email protected] * Correspondence: [email protected] (N.S.); [email protected] (E.D.) Abstract: The rapid growth of drug-resistant bacteria all over the world has given rise to a major research challenge, namely a search for alternative treatments to which bacteria will be unable to develop resistance. Photodynamic therapy is an approach of this kind. It involves the use of photo- sensitizers in combination with visible light at a certain wavelength to excite the former and generate reactive oxygen species. Various synthetic heterocyclic compounds are used as photosensitizers. Of these, derivatives of natural chlorophylls have a special place due to their properties. This review deals with the use of such compounds in antimicrobial PDT. Citation: Suvorov, N.; Pogorilyy, V.; Keywords: photodynamic therapy (PDT); photosensitizers (PS); antimicrobial PDT; chlorophyll; bac- Diachkova, E.; Vasil’ev, Y.; Mironov, teriochlorophyll A.; Grin, M. Derivatives of Natural Chlorophylls as Agents for Antimicrobial Photodynamic Therapy. Int. J. Mol. Sci. 2021, 22, 6392. 1. Introduction https://doi.org/10.3390/ijms22126392 The rapid growth of drug-resistant bacteria all over the world has given rise to a major challenge, namely a search for alternative treatments to which bacteria cannot develop Academic Editor: Robert Bucki resistance. Antibiotics widely used in the past half century are losing efficiency, and doctors increasingly adopt combined approaches to the treatment of infectious diseases. Received: 19 April 2021 Photodynamic therapy (PDT) involves the use of photosensitizers (PS) combined Accepted: 8 June 2021 Published: 15 June 2021 with light at a specific wavelength to excite the PS. The latter transfer energy to molecular oxygen. As a result, reactive oxygen species are formed, among which singlet oxygen is the Publisher’s Note: MDPI stays neutral main cytotoxic agent that can kill bacteria by oxidizing vital intracellular structures [1–4]. with regard to jurisdictional claims in Antimicrobial PDT is a relatively new method for the treatment of bacterial infections. published maps and institutional affil- This method of fighting pathogenic microorganisms has a few advantages: the ability iations. to cause localized impact on an infected site by means of local irradiation, along with high activity against Gram-positive and Gram-negative bacteria [5–7]. A photosensitizer penetrates the body and binds to a target cell, while causing no adverse effect by itself. Light is required to trigger photochemical reactions. Photosensitizers are capable of absorbing light in a strictly defined, narrow range, which is specific for each substance and is called Copyright: © 2021 by the authors. Licensee MDPI, Basel, Switzerland. the “absorption peak”. As a result, a transition of the dye molecule to an excited state This article is an open access article occurs, followed by the generation of reactive oxygen species. Different photosensitizers distributed under the terms and have a different set of absorption bands, the position and extinction coefficient of which conditions of the Creative Commons depend on the structure of the molecule. For example, for drugs of the chlorin group, the Attribution (CC BY) license (https:// absorption maximum is 660–670 nm, and for bacteriochlorins it is 100 nm more. The shift creativecommons.org/licenses/by/ of the absorption maximum to the near-IR region affects the depth of light penetration 4.0/). into the tissues. Thus, the average penetration depth of light is approximately 1–3 mm at Int. J. Mol. Sci. 2021, 22, 6392. https://doi.org/10.3390/ijms22126392 https://www.mdpi.com/journal/ijms Int. J. Mol. Sci. 2021, 22, 6392 2 of 15 630 nm and up to 10 mm at 700–850 nm [8]. The presence of endogenous chromophores (hemoglobin, melanin, etc.) leads to the absorption of the energy of the incoming light, which weakens its penetration into tissues. Therefore, the greatest “transparency” of tissues is observed at wavelengths over 780 nm [9,10]. The antimicrobial PDT has several advantages over traditional antimicrobial chemother- apy with antibiotics: it offers a short inactivation time, does not lead to the bacterial resis- tance after multi-course treatment, does not cause dysbacteriosis, and is applicable to a wide range of target microorganisms [11]. A significant advantage of antimicrobial PDT is the possibility of a target effect in the infected areas located both on the surface and in the underlying tissues, without undesirable effects on the surrounding tissues and the natural microflora. 2. Principles of Antimicrobial PDT It was shown in the 1990s that the susceptibility of Gram-positive and Gram-negative bacteria to PDT differs [12–14]. Neutral, anionic, and cationic PS molecules can efficiently bind to Gram-positive bacteria, whereas only cationic PS or neutral PS combined with membrane-permeating agents can bind to Gram-negative bacteria. An Israeli team of scientists (Y. Nitan et al.) suggested the use of the polycationic drug Polymyxin B to increase the permeability of the outer membrane of Gram-negative bacteria and allow the PS to penetrate inside them [15]. A different approach was reported by G. Bertoloni et al. [16] who found that the use of ethylenediaminetetraacetic acid also increased the permeability of the outer membrane of Gram-negative bacteria, thus facilitating the internalization of the PS. The difference in the sensitivity of bacteria to PDT is based on their structure. Gram- positive bacteria have a cytoplasmic membrane surrounded by a porous cell wall that consists of the peptidoglycan and lipoteichoic acids, which allow PS to readily bind to it. The shells of Gram-negative bacteria have the outer and inner cytoplasmic membranes separated by a layer of peptidoglycan. The outer membrane forms an efficient barrier to the permeation of many substances into a cell. The cell walls of pathogenic fungi have a thick layer of β-glucan and chitin, and they have an intermediate permeability between Gram-positive and Gram-negative bacteria. In contrast, for eukaryotic cells, the main components of membranes are neutral lipids, which leads to the absence of a charge on them [17]. Since the outer membranes of pathogenic bacteria and fungi carry a negative charge, molecules with positive charges are versatile broad-spectrum PS for antimicrobial therapy. To date, many scientific works aimed at the use of synthetic porphyrins in the PDT have been published. Their cationic derivatives show bactericidal efficiency against Gram- positive and Gram-negative bacteria in several studies [18–23]. It is relevant to use antimicrobial PDT for destruction of bacterial biofilms. The latter are organized communities of bacteria integrated into a biofilm and protected from damage by the environmental factors [24,25]. The most important property of biofilms in clinical practice is their resistance to the antibacterial drugs which is 1000 times higher than for f bacteria in a planktonic culture [26]. The resistance is associated with the violation of the interaction between the antibiotic and its target. In planktonic bacteria, this phenomenon is observed at the level of a bacterial cell that is in direct contact with a drug. There are several classic mechanism types for these bacteria associations: a target modification, the antibiotic inactivation, an active antibiotic elimination from a microbial cell (‘efflux’), an impaired permeability of microbial cell’s external structures, and the formation of a metabolic “shunt”. The development of the biofilm resistance is more complicated. Mechanisms that prevent the action of antibiotics on pathogens into biofilms include [27]: • Limited penetration of antimicrobial substances into biofilms, since the matrix slows down this process and binds antibiotics. • Differences in metabolic activities and growth rates of individual bacterial cells. Int. J. Mol. Sci. 2021, 22, 6392 3 of 15 • Restriction of nutrients and the altered microenvironment in the biofilm leading to a decrease in the rate of bacteria division and the targets number for the antibiotics ac- tion. • Enhanced mutability of bacteria in the biofilm. Under the influence of strong antibiotic therapy, the rate of mutations leading to antibiotic resistance in Pseudomonas aeruginosa biofilms can reach 20% [24,25]. • Expression of undetected resistance genes. • Interspecies transmission of genes for the antibiotic resistance, which is more success- fully implemented in conditions of close contact of bacteria inside the biofilm. • ‘Efflux pump’. One of the mechanisms of resistance is the systems of the active release (‘efflux’) of
Details
-
File Typepdf
-
Upload Time-
-
Content LanguagesEnglish
-
Upload UserAnonymous/Not logged-in
-
File Pages15 Page
-
File Size-