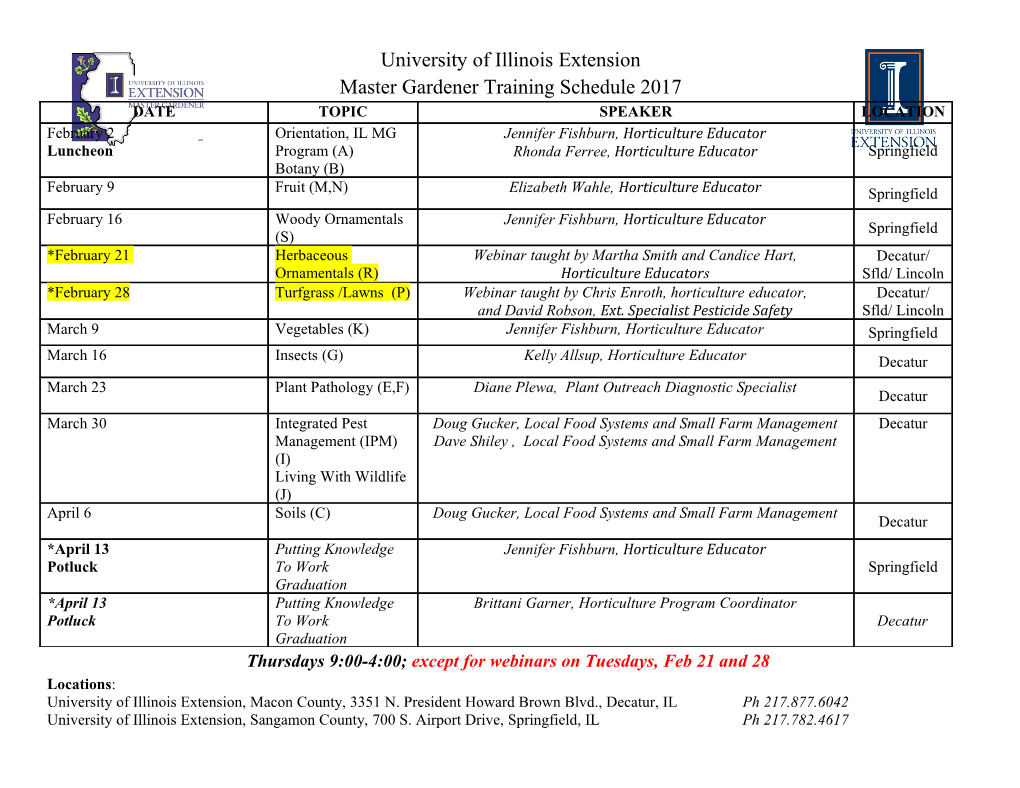
PUBLICATIONS Journal of Geophysical Research: Solid Earth RESEARCH ARTICLE Fault segmentation: New concepts from the Wasatch 10.1002/2015JB012519 Fault Zone, Utah, USA Key Points: Christopher B. DuRoss1, Stephen F. Personius1, Anthony J. Crone2, Susan S. Olig3,4, • Wasatch fault segmentation evaluated 5 6 7 via synthesis of late Holocene Michael D. Hylland , William R. Lund , and David P. Schwartz paleoearthquakes 1 2 3 • Complex ruptures shorter or longer U.S. Geological Survey, Golden, Colorado, USA, U.S. Geological Survey-Emeritus, Golden, Colorado, USA, Olig Seismic 4 than the primary segment lengths Geology, Inc., Martinez, California, USA, Formerly at URS Corporation, Seismic Hazards Group, Oakland, California, USA, are possible 5Utah Geological Survey, Salt Lake City, Utah, USA, 6Utah Geological Survey-Emeritus, Cedar City, Utah, USA, 7U.S. • Wasatch fault compared to other Geological Survey, Menlo Park, California, USA multisegment normal faults Abstract The question of whether structural segment boundaries along multisegment normal faults Correspondence to: such as the Wasatch fault zone (WFZ) act as persistent barriers to rupture is critical to seismic hazard C. B. DuRoss, analyses. We synthesized late Holocene paleoseismic data from 20 trench sites along the central WFZ to [email protected] evaluate earthquake rupture length and fault segmentation. For the youngest (<3 ka) and best-constrained earthquakes, differences in earthquake timing across prominent primary segment boundaries, especially for the Citation: most recent earthquakes on the north-central WFZ, are consistent with segment-controlled ruptures. However, DuRoss, C. B., S. F. Personius, A. J. Crone, broadly constrained earthquake times, dissimilar event times along the segments, the presence of smaller-scale S. S. Olig, M. D. Hylland, W. R. Lund, and D. P. Schwartz (2016), Fault segmentation: (subsegment) boundaries, and areas of complex faulting permit partial-segment and multisegment (e.g., New concepts from the Wasatch Fault spillover) ruptures that are shorter (~20–40km)orlonger(~60–100 km) than the primary segment lengths Zone, Utah, USA, J. Geophys. Res. Solid – – (35 59 km). We report a segmented WFZ model that includes 24 earthquakes since ~7 ka and yields mean Earth, 121,1131 1157, doi:10.1002/ – – 2015JB012519. estimates of recurrence (1.1 1.3 kyr) and vertical slip rate (1.3 2.0 mm/yr) for the segments. However, additional rupture scenarios that include segment boundary spatial uncertainties, floating earthquakes, and multisegment Received 10 SEP 2015 ruptures are necessary to fully address epistemic uncertainties in rupture length. We compare the central WFZ to Accepted 6 JAN 2016 paleoseismic and historical surface ruptures in the Basin and Range Province and central Italian Apennines Accepted article online 11 JAN 2016 fi Published online 18 FEB 2016 and conclude that displacement pro les have limited value for assessing the persistence of segment boundaries but can aid in interpreting prehistoric spillover ruptures. Our comparison also suggests that the probabilities of shorter and longer ruptures on the WFZ need to be investigated. 1. Introduction Multisegment normal faults in areas of active horizontal extension, such as the Wasatch fault zone (WFZ) in the Basin and Range Province (BRP) of the western United States, pose a conundrum for seismic hazard assess- ments: will the individual faults that linked to form these structures continue to act independently, or will they eventually form a continuous, mature fault zone that experiences throughgoing ruptures across relict structural complexities [Scholz and Gupta, 2000; Mirabella et al., 2005]? That is, are prominent structural complexities along multisegment normal faults (e.g., step overs or gaps in faulting) effective barriers to rupture [e.g., Aki, 1979; Schwartz and Coppersmith, 1984; King and Nabelek,1985;Schwartz,1989;Wesnousky, 2008], or do the faults ultimately interact, link, and bypass the complexities [e.g., Peacock and Sanderson, 1991; Willemse et al., 1996; Scholz and Gupta,2000;Chang and Smith,2002;Manighetti et al., 2007, 2015]? The question of what drives rupture initiation and termination is important to seismic hazard analyses. Controlling factors include a fault’s geometry, nearby crustal rheology, failure criterion (friction law), and initial stress conditions that stem from the history of strain accumulation and moment release on the fault, fluid pressure, and the degree of mechanical interaction and stress triggering from nearby structures [Harris, 1998, 2004]. Mechanically interacting faults are those that interact and influence each other’s distribu- tion of stresses, earthquake timing, and displacement [e.g., Peacock and Sanderson, 1991; Willemse et al., 1996; Gupta and Scholz, 2000; Scholz and Gupta, 2000]. However, evidence of mechanical interaction between faults or segments does not necessarily imply synchronous rupture, but only that the faults do not act indepen- dently. The potential for throughgoing rupture may relate to this degree of interaction [Scholz and Gupta, 2000]. Fault maturation or normal fault growth as a function of alternating periods of displacement accumu- ©2016. American Geophysical Union. lation and lateral propagation may also control the process of fault segmentation [Manighetti et al., 2015]. All Rights Reserved. Ultimately, for faults lacking historical surface-faulting earthquakes, the question of what controls rupture DUROSS ET AL. CENTRAL WASATCH FAULT ZONE SEGMENTATION 1131 Journal of Geophysical Research: Solid Earth 10.1002/2015JB012519 length is a critical one as it drives estimates of seismic moment release, earthquake recurrence, and moment magnitude for individual rupture sources [e.g., Wells and Coppersmith, 1994; Stirling et al., 2002; Manighetti et al., 2007; Wesnousky, 2008] and thus has the potential to impact local and regional probabilistic seismic hazard assessments [e.g., Working Group on California Earthquake Probabilities (WGCEP), 2003, 2008; Field et al., 2014; Petersen et al., 2014; Wong et al., 2016]. In this study, we consider the degree to which paleoseismic data can help resolve prehistoric rupture bound- aries. Even the best-constrained paleoearthquakes have timing uncertainties of tens [e.g., Scharer et al., 2007, 2014; Shennan et al., 2014] to hundreds [e.g., Lund, 2005; DuRoss, 2008] of years. Thus, it is not possible to unequivocally differentiate between synchronous rupture of two adjacent segments and the occurrence of separate earthquakes spaced closely in time (i.e., minutes to decades) [DuRoss and Hylland, 2015]. Further, although per event displacements and along-strike rupture profiles can limit rupture dimensions and magni- tudes [e.g., Hemphill-Haley and Weldon, 1999; Scholz and Gupta, 2000; Biasi and Weldon, 2006; Wesnousky, 2008; Hecker et al., 2010], the results are nonunique. For example, an anomalously large displacement near a segment boundary could be evidence of either interacting faults [e.g., Willemse et al., 1996] or natural slip variability on an isolated fault [e.g., Hecker et al., 2013]. Thus, paleoseismologists must rely on a combination of surface-fault geometry and complexity, comparison of paleoseismic earthquake-timing and displacement data, probabilistic estimates of rupture length, and historical surface-faulting analogs to quantify past rupture behavior and provide input data for seismic hazard assessments. In this paper, we examine the issues of fault segmentation and rupture length through the lens of the WFZ—one of the best-studied, multisegment normal faults in the world. Our goal is to evaluate the segmentation of the WFZ using a compilation of late Holocene earthquake-timing and displacement data for the central, most active, part of the fault, and to compare our results with those for other well-studied normal faults in extensional provinces. 1.1. The Wasatch Fault Zone The WFZ is an approximately 370 km long, multisegment normal fault that forms a prominent structural and physiographic eastern boundary (the Wasatch Front) to the ~700 km wide BRP [Gilbert, 1884, 1928; Cluff et al., 1970, 1973]. For the central WFZ (Figure 1), faulting began ~18 Ma, based on fluid inclusions in fault rock exhumed from ~11 km depth [Parry and Bruhn, 1987]. Vertical slip rates on the WFZ are higher than those for nearby normal faults west of the BRP margin [e.g., Lund, 2005] and apparently have varied through time. Slip rates based on range-front exhumation data are about ~0.7 mm/yr since ~18 Ma [Parry and Bruhn, 1987], mostly ~0.2–0.4 mm/yr since ~5 Ma [Armstrong et al., 2004] and ~0.5–1.0 mm/yr since ~0.4–0.8 Ma [Mayo et al., 2009]. In contrast, displaced late Pleistocene geomorphic surfaces yield vertical slip rates of ~0.1–0.3 mm/yr [Machette et al., 1992]. Slip rates based on latest Pleistocene to Holocene paleoseismic trenching data are ~1–2mm/yr [e.g., Machette et al., 1992; Friedrich et al., 2003; Lund, 2005]. Contemporary horizontal extension across the WFZ is ~1.6–3.2 mm/yr [Chang et al., 2006; Kreemer et al., 2010], which is about 50–90% of the strain budget for the 200 km wide eastern BRP [Dixon et al., 2000; Chang et al., 2006]. The WFZ has been divided into 10 structural segments based on along-strike changes in fault geometry, displacement, and timing of most recent movement [Swan et al., 1980; Schwartz and Coppersmith, 1984; Machette et al., 1992; Wheeler and Krystinik, 1992]. Five of these segments (from Brigham City to Nephi; herein the central WFZ; Figure 1) have
Details
-
File Typepdf
-
Upload Time-
-
Content LanguagesEnglish
-
Upload UserAnonymous/Not logged-in
-
File Pages27 Page
-
File Size-