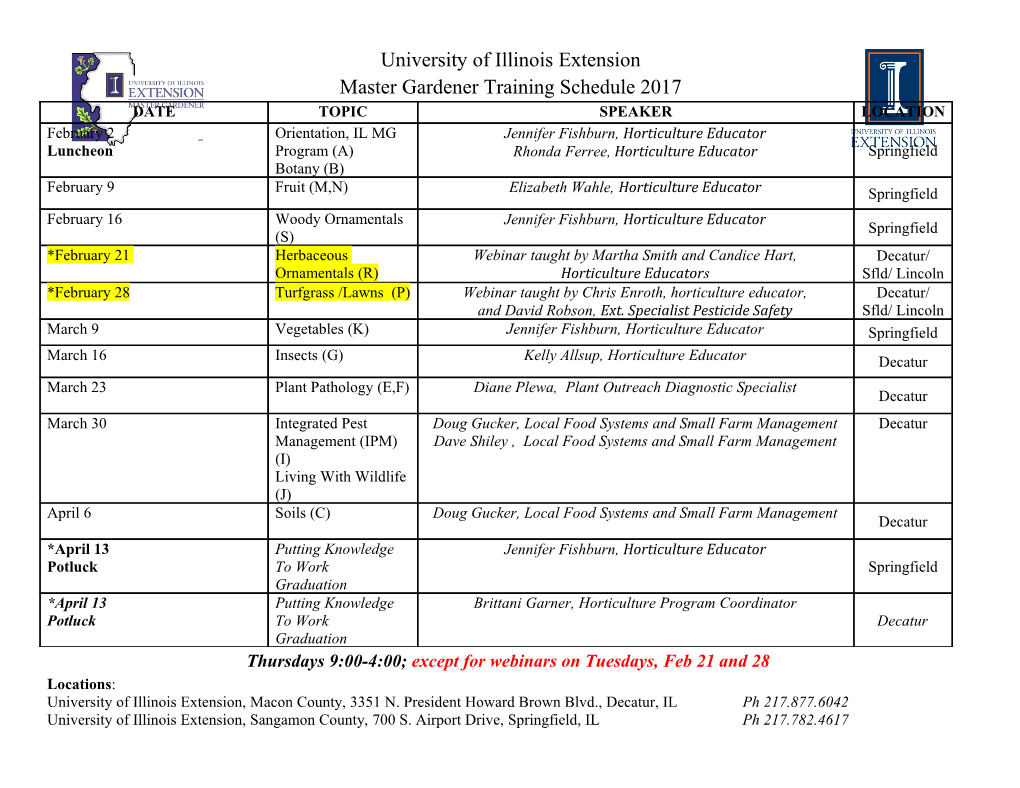
Basic & Clinical Pharmacology, 14e Chapter 21: IntroductionIntroduction toto thethe PharmacologyPharmacology ofof CNSCNS DrugsDrugs John A. Gray INTRODUCTION Drugs acting in the central nervous system (CNS) were among the first to be discovered by primitive humans and are still the most widely used group of pharmacologic agents*. These include medications used to treat a wide range of neurologic and psychiatric conditions as well as drugs that relieve pain, suppress nausea, and reduce fever, among other symptoms. In addition, many CNS-acting drugs are used without prescription to increase the sense of well-being. Due to their complexity, the mechanisms by which various drugs act in the CNS have not always been clearly understood. In recent decades, however, dramatic advances have been made in the methodology of CNS pharmacology. It is now possible to study the action of a drug on individual neurons and even single receptors within synapses. The information obtained from such studies is the basis for several major developments in studies of the CNS. First, it is clear that nearly all drugs with CNS e!ects act on specific receptors that modulate synaptic transmission. While a few agents such as general anesthetics and alcohol may have nonspecific actions on membranes (although these exceptions are not fully accepted), even these non–receptor-mediated actions result in demonstrable alterations in synaptic transmission. Second, drugs are among the most valuable tools for studying CNS function, from understanding the mechanism of convulsions to the laying down of long-term memory. Both agonists that mimic natural transmitters (and in many cases are more selective than the endogenous substances) and antagonists are extremely useful in such studies. Third, unraveling the actions of drugs with known clinical e!icacy has led to some of the most fruitful hypotheses regarding the mechanisms of disease. For example, information about the action of antipsychotic drugs on dopamine receptors has provided the basis for important hypotheses regarding the pathophysiology of schizophrenia. Studies of the e!ects of a variety of agonists and antagonists on γ-aminobutyric acid (GABA) receptors have resulted in new concepts pertaining to the pathophysiology of several diseases, including anxiety and epilepsy. A full appreciation of the e!ects of a drug on the CNS requires an understanding of the multiple levels of brain organization, from genes to circuits to behavior. This chapter provides an introduction to the functional organization of the CNS and its synaptic transmitters as a basis for understanding the actions of the drugs described in the following chapters. * The author thanks Dr. Roger A. Nicoll for his contributions to previous editions. ORGANIZATION OF THE CNS The CNS is composed of the brain and spinal cord and is responsible for integrating sensory information and generating motor output and other behaviors needed to successfully interact with the environment and enhance species survival. The human brain contains about 100 billion interconnected neurons surrounded by various supporting glial cells. Throughout the CNS, neurons are either clustered into groups called nuclei or are present in layered structures such as the cerebellum or hippocampus. Connections among neurons both within and between these clusters form the circuitry that regulates information flow through the CNS. Neurons Neurons are electrically excitable cells that process and transmit information via an electrochemical process. There are many types of neurons in the CNS, and they are classified in multiple ways: by function, by location, and by the neurotransmitter they release. The typical neuron, however, possesses a cell body (or soma) and specialized processes called dendrites and axons (Figure 21–1). Dendrites, which form highly branched complex dendritic “trees,” receive and integrate the input from other neurons and conduct this information to the cell body. The axon carries the output signal of a neuron from the cell body, sometimes over long distances. Neurons may have hundreds of dendrites but generally have only one axon, although axons may branch distally to contact multiple targets. The axon terminal makes contact with other neurons at specialized junctions called synapses where neurotransmitter chemicals are released that interact with receptors on other neurons. FIGURE 21–1 Neurons and glia in the CNS. A typical neuron has a cell body (or soma) that receives the synaptic responses from the dendritic tree. These synaptic responses are integrated at the axon initial segment, which has a high concentration of voltage-gated sodium channels. If an action potential is initiated, it propagates down the axon to the synaptic terminals, which contact other neurons. The axon of long-range projection neurons are insulated by a myelin sheath derived from specialized membrane processes of oligodendrocytes, analogous to the Schwann cells in the peripheral nervous system. Astrocytes perform supportive roles in the CNS, and their processes are closely associated with neuronal synapses. Neuroglia In addition to neurons, there are a large number of nonneuronal support cells, called glia, that perform a variety of essential functions in the CNS. Astrocytes are the most abundant cell in the brain and play homeostatic support roles, including providing metabolic nutrients to neurons and maintaining extracellular ion concentrations. In addition, astrocyte processes are closely associated with neuronal synapses where they are involved in the removal and recycling of neurotransmitters a"er release and play increasingly appreciated roles in regulating neurotransmission (see below). Oligodendrocytes are cells that wrap around the axons of projection neurons in the CNS forming the myelin sheath (Figure 21–1). Similar to the Schwann cells in peripheral neurons, the myelin sheath created by the oligodendrocytes insulates the axons and increases the speed of signal propagation. Damage to oligodendrocytes occurs in multiple sclerosis, and thus, they are a target of drug discovery e!orts. Microglia are specialized macrophages derived from the bone marrow that settle in the CNS and are the major immune defense system in the brain. The cells are actively involved in neuroinflammatory processes in many pathological states including neurodegenerative diseases. Blood-Brain Barrier The blood-brain barrier (BBB) is a protective functional separation of the circulating blood from the extracellular fluid of the CNS that limits the penetration of substances, including drugs. This separation is accomplished by the presence of tight junctions between the capillary endothelial cells as well as a surrounding layer of astrocyte end-feet. As such, to enter the CNS, drugs must either be highly hydrophobic or engage specific transport mechanisms. For example, the second-generation antihistamines cause less drowsiness because they were developed to be significantly more polar than older antihistamines, limiting their crossing of the BBB (see Chapter 16). Many nutrients, such as glucose and the essential amino acids, have specific transporters that allow them to cross the BBB. L-DOPA, a precursor of the neurotransmitter dopamine, can enter the brain using an amino acid transporter, whereas dopamine cannot cross the BBB. Thus, the orally administered drug L-DOPA, but not dopamine, can be used to boost CNS dopamine levels in the treatment of Parkinson’s disease. Some parts of the brain, the so-called circumventricular organs, lack a normal BBB. These include regions that sample the blood, such as the area postrema vomiting center, and regions that secrete neurohormones into the circulation. ION CHANNELS & NEUROTRANSMITTER RECEPTORS The membranes of neurons contain two types of channels defined on the basis of the mechanisms controlling their gating (opening and closing): voltage-gated and ligand-gatedligand-gated channels (Figure 21–2A and B). Voltage-gated channels respond to changes in the membrane potential of the cell. The voltage-gated sodium channel described in Chapter 14 for the heart is an example of this type of channel. In nerve cells, these channels are highly concentrated on the initial segment of the axon (Figure 21–1), which initiates the all-or-nothing fast action potential, and along the length of the axon where they propagate the action potential to the nerve terminal. There are also many types of voltage-sensitive calcium and potassium channels on the cell body, dendrites, and initial segment, which act on a much slower time scale and modulate the rate at which the neuron discharges. For example, some types of potassium channels opened by depolarization of the cell result in slowing of further depolarization and act as a brake to limit further action potential discharge. Plant and animal toxins that target various voltage-gated ion channels have been invaluable for studying the functions of these channels (see Box: Natural Toxins: Tools for Characterizing Ion Channels; Table 21–1). FIGURE 21–2 Types of ion channels and neurotransmitter receptors in the CNS. A shows a voltage-gated channel in which a voltage sensor component of the protein controls the gating (broken arrow) of the channel. B shows a ligand-gated channel in which the binding of the neurotransmitter to the ionotropic channel receptor controls the gating (broken arrow) of the channel. C shows a G protein-coupled (metabotropic) receptor, which, when bound, activates a heterotrimeric G protein. D and E show two ways metabotropic
Details
-
File Typepdf
-
Upload Time-
-
Content LanguagesEnglish
-
Upload UserAnonymous/Not logged-in
-
File Pages18 Page
-
File Size-