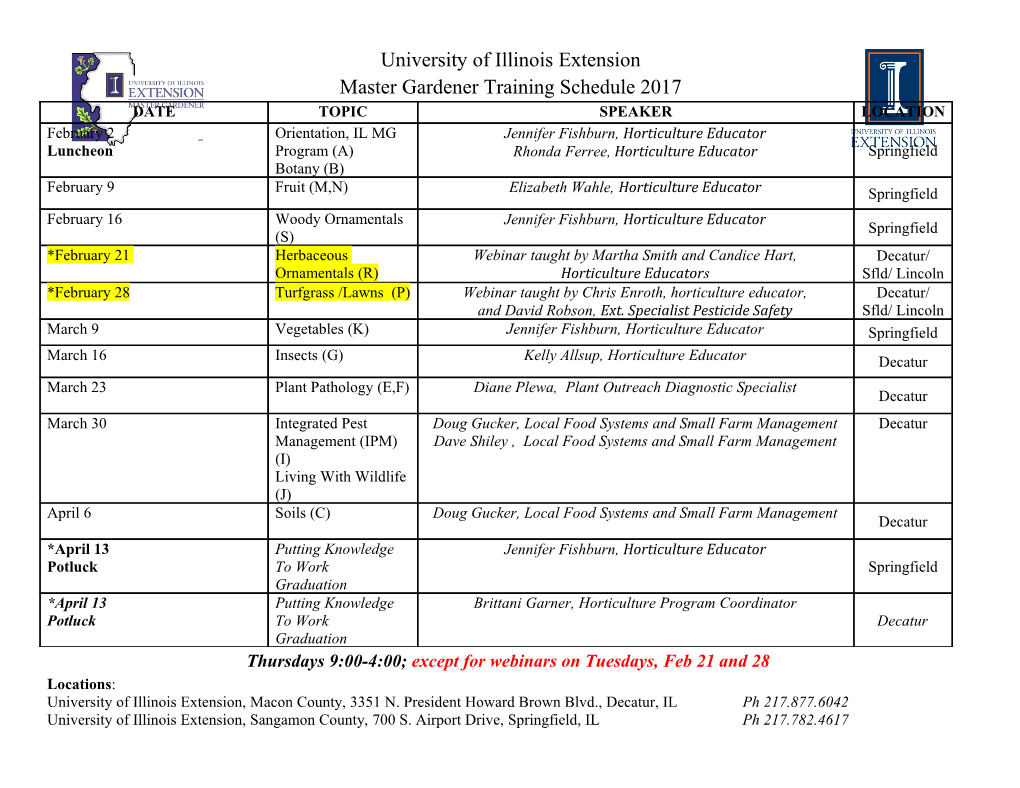
Executive Summary of Recent Utah Lake Reports: (Richards and Miller 2019, Williams 2019, Miller 2019 and Merritt 2019) Prepared by Theron G. Miller, PhD And David C. Richards, PhD January 3, 2020 Introduction This summary is intended to provide important highlights and an integration of recent studies focused on water column loading of total and soluble P and nitrogen to the water column. Sources include P and N loading from tributaries, POTW discharges, sediment recycling and atmospheric deposition. Resent results of mass balance modelling and predictive eutrophication status based on P loading are also included. Assessing the ecological integrity of Utah Lake in relation to anthropogenic forces, invasive species, current trophic status and the potential for recovery are also discussed. Reports that are summarized include: Richards and Miller 2019, Williams 2019, Miller 2019 and Merritt 2019. Physical setting While one of the largest freshwater lakes in the western US (93,000 acres), Utah Lake is a tiny remnant of ancient Lake Bonneville. Since about 1850, tributary flows have been largely diverted for agricultural and municipal use, vastly reducing the flushing rate (approximately 1 volume replacement every 2 years under normal runoff) and with approximately 4 ft of evaporation each year (52% of the lake volume), the lake is continuously slightly saline. Along with overfishing of endemic fishes, introduction of invasive species, rapid urbanization of the Utah Valley, these large alterations have rapidly shifted Utah Lake to an altered stable state that includes shallow, warm water, dominance of the benthivorous carp, elevated turbidity, loss of critically important filter feeding mollusks and other invertebrates and frequent cyanobacterial blooms (cyanoHABs). Utah Lake was in a natural mesotrophic to eutrophic state as a result of being a quasi terminal lake that receives elevated P concentrations in tributaries that flow through P-rich sedimentary bedrock. Elevated P concentrations have been further exacerbated by myriad landscape disturbances associated with urbanization, such as stormwater and wastewater discharges, agricultural development and runoff, and increasing loadings of P-rich dusts from nearby west and southern desert playas from continued water diversions, a drying climate and intensive agricultural practices. Sources of P Utah Lake is unique in that the majority of nutrient inflows remain in the Lake; with estimates that over 90% of nutrients carried by tributary streams are captured and remain in the Lake. Total nutrient inflows to UL includes streams, wastewater treatment plants, overland flow and additional nutrient reservoirs including sediment, soils, atmospheric deposition (dust and wet deposition), and biological sources (e.g., carp). Major tributaries drain large watershed areas that are comprised of ancient sedimentary formations (e.g. the Park City Formation), that are naturally rich in P and other limestone minerals. These P-rich tributaries predispose Utah Lake to elevated water column P concentrations and recent measurements of sediment P have documented very high total P and soluble P in the pore water. Further evidence of the transport of these P-rich sediments was documented in the delta sediments of Deer Creek Reservoir. Filled in 1940, sediments of the Provo River delta are relatively recent but have similar P concentrations as sediments in Utah Lake. Sediment P concentrations in Utah Lake ranged from 280 to 1710 mg/kg dry weight with an average of 666 mg/kg. Phosphorus concentrations have been found to be similar between Utah Lake sediments and soils that were collected generally within a few 100 meters of the shoreline. While soil samples may not represent recent sediment deposition and concentrations, they do likely represent dust deposited from near and far field playas and disturbed agricultural lands that are reaching Utah Lake and contribute to both the nutrient and sediment load to the lake. Two studies have evaluated the bioavailability of P in Utah lake sediments. The pore water and loosely bound (treated with NH4Cl), represented 40 to 60% of 10 random sediment samples (Randall et al. 2019). Some samples included as much 5 mg/L SRP in the pore water. While not a part of the present study, Hogsett, (2019) measured sediment P flux in SOD chambers designed to seal to the lake bottom during incubation. They reported a lake-wide estimate of 1500 metric tons of SRP released to the water column annually. Randall, et al. 2019 noted that P concentrations in the UL water column are likely governed by sediment P sources, rather than by inflows into the lake. Modeling the Nutrient Budget, and contribution from Wastewater Treatment Plants Dr. LaVere Merritt, BYU Professor Emeritus, calibrated two models, the LKSIM model, which is primarily a mass balance model of water and solutes that enter the lake, and the Larsen Mersier model, which is a modification of the Vollenweider (1975) eutrophication model. Data was obtained from a comprehensive sampling program, including flow, P, N and other solute concentrations, including POTW “end of pipe” measurements collected during a joint UDWQ- Central Utah Water Conservation District sampling effort from 2009 to 2013. Additional data sets since that time include the same and some additional sites with efforts from the WFWQC and US Bureau of Reclamation (Table 1). The reduction in P load, primarily starting in 2014 is primarily due to load reductions from upgraded Timpanogos Special Service District Water Reclamation Facility and the Orem City Water Reclamation Facility to include biological nutrient removal. Both of these plants were brought online in 2013 and the POTW contribution has recently been estimated to be 120 tons/yr. Dr. Merritt’s annual loading results are listed in Table 1. Table 1. Utah Lake Nutrient Loadings1 – 10 yr, 2009 – 2018 period – w/o Atmos. Dep2. Total P Soluble Reactive P Dis.Inorg. N Water Yr. Ton/yr Ton/yr Ton/yr 2009 264 222 2116 2010 246 210 1886 2011 309 256 2710 2012 237 202 1719 2013 240 206 1715 2014 232 198 1663 2015 227 196 1551 2016 165 132 1446 2017 199 157 1889 2018 169 135 1476 Avg 229 191 1815 _______________________________________________________________ 1With modified nutrient removal at the Timpanogos and Orem WWTPs after 2015. Using mass balance results, the P retention coefficient of Utah Lake is about 0.9, or about 90% of all P entering the lake from tributary flow is retained. Evaluation of similar eutrophic lakes and hydrologic retention times suggests an average retention coefficient of 0.5 would be normal (Figure 1). Merritt suggests that this discrepancy supports the notion of ongoing chemical precipitation of P with various minerals, including carbonate-minerals such as apatite or redox-sensitive complexes with Fe, Al, Mn and Mg - a process that is just now beginning to receive necessary attention. For example, redox sensitive compounds are likely responsible for the elevated soluble P found in pore water samples (Randall 2019) and the net sediment efflux of P to the water column reported by Hogsett et al. (2019). This supports the conclusion of Randall (2019) where it is noted that sediment chemistry and nutrient reflux plays a major role in the nutrient budget of Utah Lake. As mentioned above, Merritt’s current use of the Larsen-Mercier model includes only tributary inflow and a P retention coefficient of only 0.5 (Figure 1). This represents a very conservative estimate of P loadings and retention. While this seems like a minor curiosity, it does not consider additional loadings from atmospheric deposition nor extremely large contribution of P to the water column from sediments. Briefly, even if 100% of P was removed from POTW discharges, plus an additional 25% removal from other tributary and nonpoint sources, the model predicts that an average of 90 ug/L would remain in tributary contributions, an amount sufficient to retain a eutrophic condition in Utah Lake. WWTP TP removal µg/l 697 None 523 Orem/Timp 1mg/l 307 All at 1 mg/l 107 All at 0.1 mg/l 90 Plus 25% out of all other Fig. 1. Larsen-Mercier Trophic State Model for Utah Lake (w/o AD) Dr. Merritt further noted that “in-situ concentrations of TP in the lake appear to have not changed since appreciable lake sampling began some 30 years ago, while during this period (w/o AD) the TP loadings increased perhaps 40% over that time period to 2016 and now have dropped back to about the same as 30 years ago with Phase 1 TP removal at the Timpanogos and Orem WWTPs. The long-term monthly summer averages of TP values along the middle of the main lake have remained mostly in the 50 ug/l to 70 ug/l range during this period and the outflowing Jordan River TP concentrations have been essentially constant at an avg. of about 50 ug/l. This indicates Lake TP retention (largely precipitated) in the 2018 water year was 89% (w/o AD) and 95% (w AD). Additional evidence of TP precipitation of any amount added above about 50 to 60 ug/l TP is shown in Fig. 2. This plot shows that the amount of algal growth (as indexed by Chlorophyll-a) is independent of the external TP loadings”. 120 ] 100 y = -0.1603x + 34.121 80 R² = 0.0051 a [ug/l - 60 40 Chlorophyll 20 0 0.00 5.00 10.00 15.00 20.00 25.00 30.00 35.00 40.00 LKSIM: Total-Phosphors Loadings [tons/mon] Fig. 2. Utah Lake TP Loads – Chl-a for 29 yrs (>10 & < 60) – July-Sept mon. avgs. 1990 -2018. Atmospheric Deposition Williams has summarized the two studies performed by graduate students of the BYU Engineering Department: Academic studies have focused on long-range dust transport and the NADP has developed a set of guidelines to locate sampling stations at positions that are not influenced by shortrange atmospheric transport.
Details
-
File Typepdf
-
Upload Time-
-
Content LanguagesEnglish
-
Upload UserAnonymous/Not logged-in
-
File Pages370 Page
-
File Size-