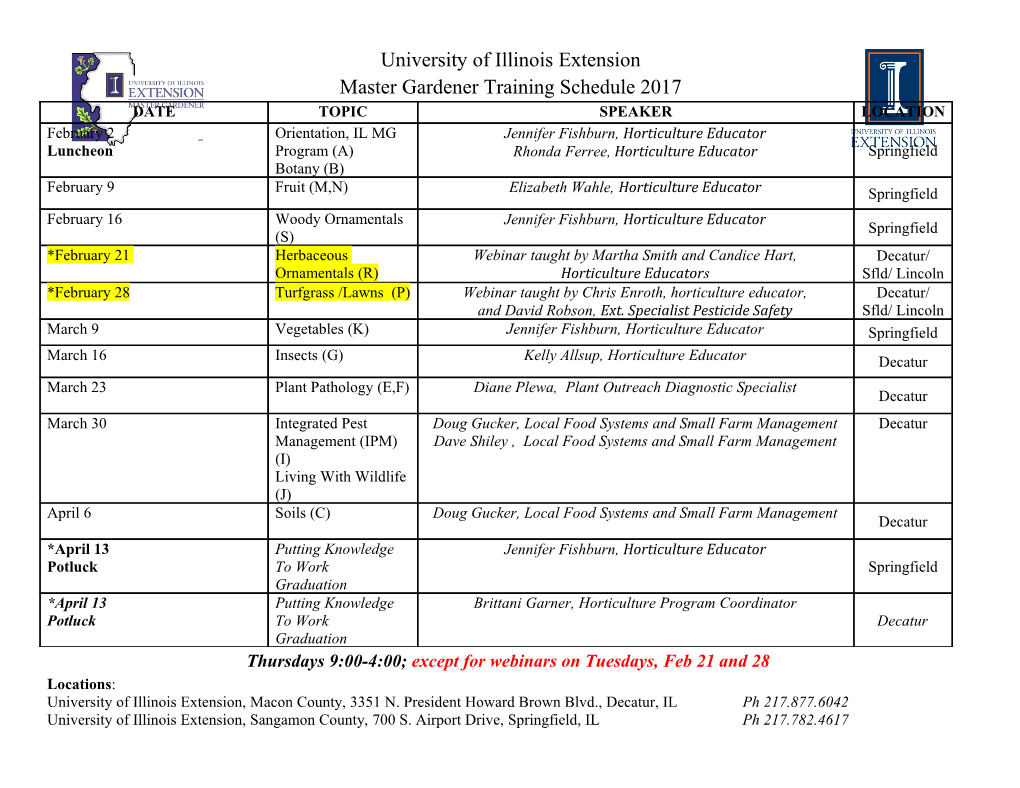
Dedicated to my parents List of Papers This thesis is based on the following papers. I Remarkably Stable Silicon Analogues of Amide Enolates: Synthesis, Structural Characterization, and Reactivity Studies. Tamaz Guliash- vili, Arnaud Martel, Alvi Muhammad Rouf, Andreas Fischer, Patrik Akselsson, and Henrik Ottosson, Manuscript. II Formation and Fundamental Properties of Potassium Germen-2- olates. Julius Tibbelin, Alvi Muhammad Rouf, Magnus Björklund, Hui Tong, Jochen Lach, and Henrik Ottosson, Manuscript. III A Computational Investigation of Brook-type Sila(hetero)aromatics and Their Possible Formation through [1,3]-Si O Silyl Shifts. Alvi Muhammad Rouf and Henrik Ottosson, Submitted. IV Silaphenolates and Silaphenylthiolates: Two Unexplored Unsaturated Silicon Compound Classes Influenced by Aromaticity. Alvi Muham- mad Rouf and Henrik Ottosson, Molecules 2012, 17, 369-389. V The [1,3]-Si O Silyl Shift from a Nonconducting Acylsilane to a Conducting Brook-Silene as Basis for a Molecular Switch. Henrik Löfås, Alvi Muhammad Rouf, Anton Grigoriev, Rajeev Ahuja, and Henrik Ottosson, preliminary manuscript. VI Highly Efficient and Convenient Acid Catalyzed Hypersilyl Protection of Alcohols and Thiols by Tris(trimethylsilyl)silyl-N,N- dimethylmethaneamide. Alvi Muhammad Rouf, Burkhard O. Jahn, Julius Tibbelin, Judith Baumgartner, Cesar Pay Gómez, and Henrik Ottosson, Submitted. VII Scope and Limitations of an Acid Catalyzed Protocol for Hypersilyl Protection of Alcohols and Thiols. Alvi Muhammad Rouf, Saithalavi Anas, Rikard Emanuelsson, Kaitlin Lozinski, and Henrik Ottosson, Manuscript. Reprints were made with permission from the respective publishers. Author Contribution The Author wishes to clarify his contribution to the included paper I Performed computational studies of NMR chemical shifts and charge distributions, as well as computational studies of the degradation mechanism of N-phenyl substituted 2-aminosilen-2-olate. II Equal participation in experimental part by first three authors. Partic- ipated in manuscript writing. III Performed all computational work and contributed extensively to manuscript writing. IV Performed all computational work and contributed partly to manu- script writing. V Performed quantum chemical calculations on isolated molecules and participated in project formulation. VI Partly formulated the project and performed all experimental work and contributed extensively to manuscript writing. VII Partly formulated the project, performed most of the experimental work, and participated in manuscript writing. Contents 1. Introduction ............................................................................................... 11 2. Theoretical and Computational Background ............................................ 15 2.1. Qualitative Bonding Models ............................................................. 15 2.2. Computational Quantum Chemical Methods .................................... 16 3. Potassium Silenolates and Germenolates .................................................. 17 3.1. An Overview of Metal Silenolates .................................................... 17 3.2. Potassium Aminosilenolates .............................................................. 20 3.2.1. Formation and Structural Properties .......................................... 20 3.2.2. Stability and Reactivity .............................................................. 21 3.2.3. Degradation of Potassium 2-diphenylaminosilen-2-olate .......... 22 3.3. Potassium Germenolates compared to Potassium Silenolates ........... 24 3.3.1. Formation of Tris(trimethylsilyl)acyl- and carbamylgermanes, and Potassium Germenolates ............................................................... 24 3.3.2. Spectroscopic and Structural Aspects ........................................ 26 3.3.3. Reactivity Studies ...................................................................... 27 4. Silaaromatics: Neutral and Anionic .......................................................... 29 4.1. Neutral Silaaromatics ........................................................................ 29 4.1.1. Historical Overview ................................................................... 29 4.1.2. Brook-type Silaaromatics .......................................................... 32 4.1.3. Silabenzenes and Silapyridine: Energy Profiles ........................ 33 4.2.3. Silabenzenes and Silapyridine: Structural Properties ................ 36 4.1.4. Silabenzenes: Stability towards Dimerization ........................... 37 4.2. Anionic Silaaromatics: Silaphenolates .............................................. 38 4.2.1. Relative Isomer Energies of Silaphenolates .............................. 39 4.2.2. Geometries of Silaphenolates .................................................... 40 4.2.3. Distribution of Charges in Silaphenolates ................................. 42 4.2.4. Nucleus independent chemical shifts (NICS) of silaphenolates 43 4.2.5. Stability of Silaphenolates towards Dimerization ..................... 44 5. Acylsilane/Brook-Silene Switches in Molecular Electronics ................... 46 5.1. Introduction ....................................................................................... 46 5.2. Acylsilane/Brook-silene Molecular Switch ....................................... 47 6. A New Acid-Catalyzed Protocol for Hypersilyl Alcohol Protection ........ 50 6.1. Introduction to the Hypersilyl Group ................................................ 50 6.2. An Acid-Catalyzed Protocol for Hypersilylether Formation ............ 51 6.2.1. Hypersilylation of Alcohols ....................................................... 52 6.2.3. Protection of Thiols ................................................................... 53 6.2.2. Selectivity in Protection of Diols ............................................... 54 6.2.4. Functional Group Tolerance ...................................................... 55 6.2.5. Proposed Mechanism of Alcohol Protection ............................. 57 6.2.6. Deprotection Protocol ................................................................ 58 7. Summary and Outlook .............................................................................. 59 8. Summary in Swedish ................................................................................ 62 9. Summary in Urdu ...................................................................................... 66 10. Acknowledgements ................................................................................. 70 11. References ............................................................................................... 71 Abbreviations Å Ångstrom Ad Adamantyl AO Atomic orbital B3LYP Becke’s three-parameter hybrid DFT method Bbt 2,6-bis[bis(trimethyl)methyl]-4- [tris(trimethylsilyl)methyl]phenyl CCSD Coupled cluster theory cp Cyclopentane DFT Density functional theory DMAP 4-dimethylaminopyridine E Energy e Electron charge Et Ethyl eq Equivalent eqn. Equation iPr Isopropyl IR Infrared (irradiation) h or hrs Hour(s) M062X The Minnesota 06 hybrid meta DFT functional of Truhlar MADs Mean absolute deviations Me Methyl MeI Methyl iodide min Minute nBu Normal butyl N(-CH2-) Piperidyl NICS Nucleus independent chemical shift NMR Nuclear magnetic resonance NPA Natural population analysis OTMS Trimethylsiloxy ppm Parts per million pri Primary r.t. Room temperature sec Secondary TBS tert-butyldimethylsilyl Tbt 2,4,6-tris[bis(trimethylsilyl)methyl]phenyl tBu Tertiary butyl tBuOK Potassium tert-butoxide tert Tertiary TIPS triisopropylsilyl TFA Trifluoroacetic acid TfOH Triflic acid (trifluoromethane sulfonic acid) TsOH Tosylic acid (para-toluene sulfonic acid) THF Tetrahydrofurane TMS Trimethylsilyl UV Ultraviolet 1. Introduction Silicon comprises approximately 28% of Earth’s crust and it is the second most abundant element after oxygen. However, its uses in organic chemistry are limited when compared with carbon. The major everyday applications of silicon chemistry lie in the form of organosilicon polymers known as sili- cones.1 Within organic synthesis, organosilanes have also found extensive use in the protection of functional groups because of their facile addition and removal.2 Due to their low toxicity, the demand for the preparation of sili- con-based bioactive molecules is also gaining interest.1,3 Throughout time, unsaturated compounds of Si and the heavier Group 14 elements (Ge, Sn, and Pd) have received less interest because of difficulties in their syntheses and in the study of their properties.4,5 Although organosili- con chemistry has been known for more than 100 years,6 the first successful formation of a transient Si=C double bonded compound, a so-called silene, was reported by Guselnikov and Flowers in 1967.7 The high reactivity is a major problem in the isolation and characterization of these species, and mostly they have been isolated as [2+2] dimers or as products with various trapping reagents. However, in 1981 Brook and co-workers formed the first isolable silene 1-2 by a photochemical [1,3]-Si O shift of a trimethylsilyl (TMS) group from a tetrahedral silicon atom to an adjacent carbonyl oxygen of acylpolysilane 1-1 (Scheme 1.1).8 The reaction can also be performed thermally, and until today silenes have been generated by this route at tem- peratures in the range 65 – 250 oC.9-12 Scheme 1.1: The photochemically or thermally induced [1,3]-TMS shift from sili- con to oxygen. Influence of reverse Si=C bond polarization (Si- =C+ (II) vs.
Details
-
File Typepdf
-
Upload Time-
-
Content LanguagesEnglish
-
Upload UserAnonymous/Not logged-in
-
File Pages76 Page
-
File Size-