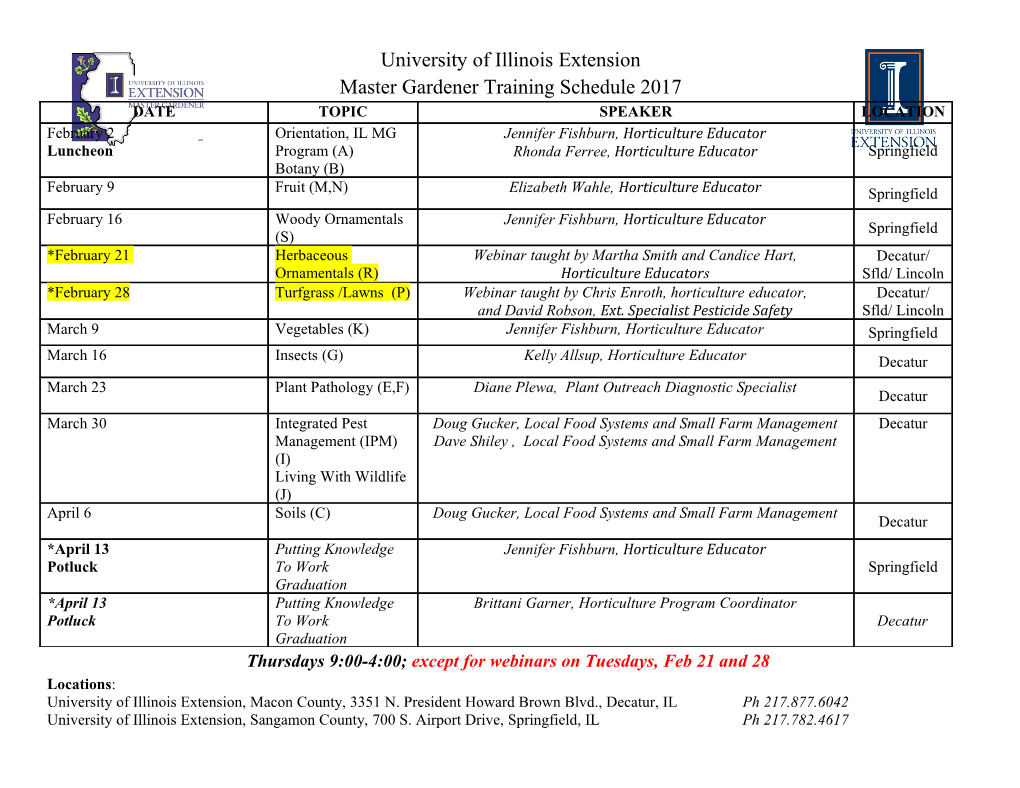
Chapter 2 Methionine Aminopeptidase Emerging role in angiogenesis Joseph A. Vetro1, Benjamin Dummitt2, and Yie-Hwa Chang2 1Department of Pharmaceutical Chemistry, University of Kansas, 2095 Constant Ave., Lawrence, KS 66047, USA. 2Edward A. Doisy Department of Biochemistry and Molecular Biology, St. Louis University Health Sciences Center, 1402 S. Grand Blvd., St. Louis, MO 63104, USA. Abstract: Angiogenesis, the formation of new blood vessels from existing vasculature, is a key factor in a number of vascular-related pathologies such as the metastasis and growth of solid tumors. Thus, the inhibition of angiogenesis has great potential as a therapeutic modality in the treatment of cancer and other vascular-related diseases. Recent evidence suggests that the inhibition of mammalian methionine aminopeptidase type 2 (MetAP2) catalytic activity in vascular endothelial cells plays an essential role in the pharmacological activity of the most potent small molecule angiogenesis inhibitors discovered to date, the fumagillin class. Methionine aminopeptidase (MetAP, EC 3.4.11.18) catalyzes the non-processive, co-translational hydrolysis of initiator N-terminal methionine when the second residue of the nascent polypeptide is small and uncharged. Initiator Met removal is a ubiquitous and essential modification. Indirect evidence suggests that removal of initiator Met by MetAP is important for the normal function of many proteins involved in DNA repair, signal transduction, cell transformation, secretory vesicle trafficking, and viral capsid assembly and infection. Currently, much effort is focused on understanding the essential nature of methionine aminopeptidase activity and elucidating the role of methionine aminopeptidase type 2 catalytic activity in angiogenesis. In this chapter, we give an overview of the MetAP proteins, outline the importance of initiator Met hydrolysis, and discuss the possible mechanism(s) through which MetAP2 inhibition by the fumagillin class of angiogenesis inhibitors leads to cytostatic growth arrest in vascular endothelial cells. Key words: methionine aminopeptidase, angiogenesis, TNP-470, AGM-1470, ovalicin, vascular endothelial cells, protein turnover. Aminopeptidases in Biology and Disease, Edited by Hooper and Lendeckel, Kluwer Academic/Plenum Publishers, New York, 2004 17 18 VETRO, DUMMITT, AND CHANG Chapter 2 1. CO-TRANSLATIONAL HYDROLYSIS OF INITIATOR METHIONINE 1.1 Overview Eubacteria (Adams and Capecchi 1966; Clark and Marcker 1966), as well as mitochondria (Bianchetti et al. 1977) and chloroplasts (Lucchini and Bianchetti 1980) which are probably the descendants of endosymbiotic eubacteria (Gray 1992), initiate mRNA translation with an Nα-formylated Met methionine bound to an initiator tRNA (f-Met-tRNAf ) (Leder and Bursztyn 1966; Noll 1966). Eukaryotes (Housman et al. 1970) and archaebacteria (Ramesh and RajBhandary 2001) initiate the translation of Met cytosolic mRNA with a methionine-bound initiator tRNA (Met-tRNAi ). As a result, the primary structure of the majority of all nascent polypeptides, with the exception of a small number of proteins that initiate translation from rare non-AUG codons, begins with an initiating Nα-formylated N-terminal methionine (f-Metinit) or N-terminal methionine (Metinit). Metinit is co-translationally hydrolyzed in a non-processive manner by methionine aminopeptidase (MetAP, E.C. 3.4.11.18, product of the MAP gene) when the second residue in the primary structure of the nascent polypeptide is small and uncharged (Ala, Cys, Gly, Pro, Ser, Thr, Val) (Adams 1968; Ben-Bassat et al. 1987; Boissel et al. 1985; Flinta et al. 1986; Huang et al. 1987; Tsunasawa et al. 1985). Metinit hydrolysis in eubacteria, however, only occurs after deformylation of f-Metinit (Adams 1968) as MetAP activity requires a free α-amino group (Solbiati et al. 1999). It is estimated that approximately 60 to 64% of mature proteins in Escherichia coli have Metinit removed (Hirel et al. 1989; Waller 1963). A similar survey in animal cells suggests that approximately 70% of mature proteins have Metinit removed (Boissel et al. 1985). The hydrolysis of Metinit by MetAP is part of a series of possible co- translational N-terminal modifications (Figure 1). In eubacteria, the formyl group of f-Metinit is first removed from all nascent polypeptides by a peptide deformylase (PDF). Metinit is then hydrolyzed by MetAP when the nascent polypeptide is between 40 and 50 amino acids in length (Housman et al. 1972). In eukaryotes, Metinit is hydrolyzed when the nascent polypeptide is approximately 15 to 20 amino acids in length (Jackson and Hunter 1970). Metinit hydrolysis may then be followed by two secondary co-translational N- terminal modifications, Nα-acetylation (Ac) and Nα-myristoylation (Myr). These modifications occur when the nascent polypeptide is between 40 and 70 amino acids in the case of Nα-acetylation (Pestana and Pitot 1975a) or less than 100 amino acids in length in the case of Nα-myristoylation (Deichaite et Methionine Aminopeptidase 19 al. 1988). Like the removal of Metinit by MetAP, the activities of the enzymes involved in these secondary modifications (Nα-myristoyltransferase [NMT] and Nα-acetyltransferase [NAT]) are predominantly governed by the primary structure of the nascent polypeptide, but additionally rely on the timely co-translational removal of Metinit to reveal the N-terminal substrate (Boutin 1997; Polevoda and Sherman 2000). Figure 1. Summary of possible co-translational N-terminal modifications. Co-translational N- terminal modifications are defined as those that occur during polypeptide synthesis on the ribosomes and are largely governed by the primary sequence of the nascent polypeptide’s N- terminus (i.e., Metinit-AA2-AA3- where AAn is amino acid in position n). The minimal substrate requirements for the activity of each N-terminal modifying enzyme are summarized below the resulting N-terminal modification. Most mature proteins have some N-terminal modification. 1.2 Compartmentalization of Metinit and f-Metinit Hydrolysis in Higher Eukaryotes α It has been proposed that formylated Metinit is only hydrolyzed from N - formylated proteins synthesized in eubacteria and not from Nα-formylated proteins synthesized within mitochondria and chloroplasts (Mazel et al. 1994). This idea is based on the absence of a PDF gene in the genomes of Saccharomyces cerevisiae and Caenorhabditis elegans (Giglione et al. 2000b) as well as the genomes of mitochondria and chloroplasts. It was once 20 VETRO, DUMMITT, AND CHANG Chapter 2 assumed that, since eukaryotes do not possess a PDF, there would be no deformylation of f-Metinit and consequently no subsequent hydrolysis of Metinit by MetAP from proteins synthesized within mitochondria or chloroplasts (Giglione et al. 2000a). There is recent evidence, however, that both forms of Metinit hydrolysis are common in higher eukaryotes; Metinit hydrolysis takes place in the cytosol, and f-Metinit hydrolysis takes place in chloroplasts and mitochondria (Giglione et al. 2000b). 2. METHIONINE AMINOPEPTIDASES 2.1 MetAP Isoforms Two major classes of MetAPs, designated type 1 and type 2 (MetAP1 and MetAP2), were originally identified as cytosolic proteins (Arfin et al. 1995; Li and Chang 1995). The two classes are structurally similar but share little sequence homology (Lowther and Matthews 2000). Eubacteria express only a type 1 MetAP (Chang et al. 1989), whereas archaebacteria express only a type 2 MetAP (Arfin et al. 1995; Li and Chang 1995). It has recently been shown, however, that the genome of the cyanobacterium, Synechocystis sp., has both MAP1 and MAP2 genes, as well as a novel MAP3 gene (Atanassova et al. 2003). Interestingly, all eukaryotes examined to date possess cytosolic forms of both MetAP1 and MetAP2 (Arfin et al. 1995; Li and Chang 1995). Multiple isoforms of MetAP1 were also recently found to localize to the chloroplasts (MetAP1B, MetAP1C, MetAP1D) and mitochondria (MetAP1C and MetAP1D) in the plant Arabidopsis thaliana (Giglione et al. 2000b). Although likely, it has not been demonstrated whether there are MetAP isoforms that localize to mitochondria of all eukaryotes. The distribution and variety of MetAPs are more complex than originally thought. 2.2 General Structural Features of MetAP All MetAPs share a conserved C-terminal catalytic domain (Figure 2). Within the catalytic domain are five conserved amino acids that bind up to two metal ion cofactors. In Escherichia coli these five residues are Asp97, Asp108, His171, Glu204, and Glu235 (Roderick and Matthews 1993). Dialysis / flame emission analysis of MetAP1 from yeast (Saccharomyces cerevisiae) (Klinkenberg et al. 1997) and electron spin resonance analysis (Copik et al. 2003; D'souza et al. 2000) of MetAP from bacteria (Escherichia coli) Methionine Aminopeptidase 21 suggest that the second metal ion binding site is only partially occupied in MetAP1. X-ray crystal structure studies of MetAP from Escherichia coli (a type 1 MetAP) (Roderick and Matthews 1993), Pyrococcus furiosus (a type 2 MetAP) (Tahirov et al. 1998), and human MetAP2 (Liu et al. 1998) show that both type 1 and type 2 MetAPs exhibit a somewhat comparable “pita bread” symmetry (Roderick and Matthews 1993) in which both halves of the enzyme are structurally similar, although they share little sequence homology (for review see Lowther and Matthews 2000). A substrate binding pocket adjacent to the metal binding center capable of accommodating up to two amino acids has also been identified in both MetAP1 and MetAP2 (Liu
Details
-
File Typepdf
-
Upload Time-
-
Content LanguagesEnglish
-
Upload UserAnonymous/Not logged-in
-
File Pages28 Page
-
File Size-