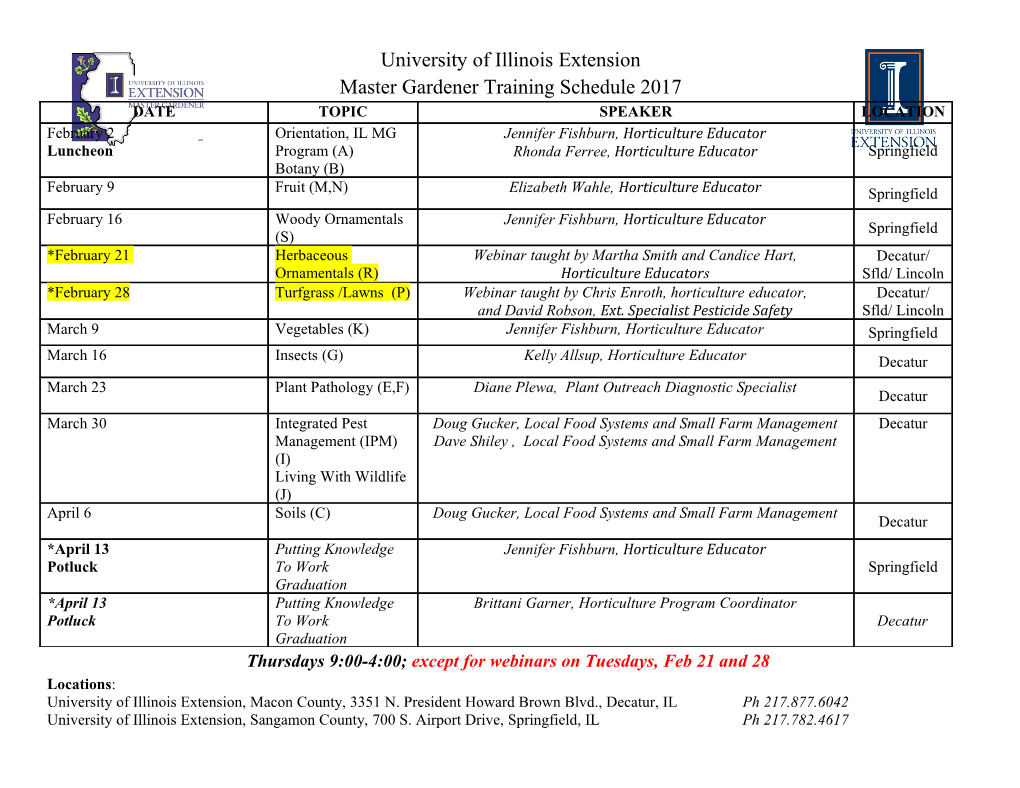
catalysts Article Economy Assessment for the Chiral Amine Production with Comparison of Reductive Amination and Transamination Routes by Multi-Enzyme System Heyu Huo 1, Guangxiao Yao 1 and Shizhen Wang 1,2,* 1 Department of Chemical and Biochemical Engineering, College of Chemistry and Chemical Engineering, Xiamen University, Xiamen 361005, China; [email protected] (H.H.); [email protected] (G.Y.) 2 The Key Lab for Synthetic Biotechnology of Xiamen City, Xiamen University, Xiamen 361005, China * Correspondence: [email protected]; Tel.: +86-592-218-3751 Received: 22 October 2020; Accepted: 6 December 2020; Published: 11 December 2020 Abstract: Chiral amines are key building blocks for pharmaceuticals. Economic assessment of commercial potential of bioprocesses is needed for guiding research. Biosynthesis of (S)-α-methylbenzylamine (MBA) was selected as case study. For transamination route, transaminase coupled with glucose dehydrogenase and lactate dehydrogenase catalyzed the reaction with NADH (Nicotinamide adenine dinucleotide) regeneration. Amine dehydrogenase coupled with NADH oxidase, which catalyzed the reductive amination process. Comparison of biosynthesis cost by reductive amination and transamination routes was carried out. Economic assessment based on the framework of cost analysis and preliminary process information revealed that cost is greatly dependent on enzyme price. The results indicated that enhancing the activity of amine dehydrogenase by 4–5 folds can drop the unit price of reductive amination to $0.5–0.6/g, which make it competitive with transamination route. Keywords: chiral amine; amine dehydrogenase; transaminase; multi-enzyme system; economic estimation; bioprocess 1. Introduction Chiral amines are key building blocks for many new pharmaceutical products. Transaminases have emerged as an attractive alternative for chiral amine synthesis, due to their high enantioselectivity. However, there are still many challenges that need to be overcome to make the technology more widely applicable such as unfavorable equilibrium, low substrate solubility, low activity, low stability of the biocatalyst as well as product, and substrate inhibition [1]. The success of a biocatalytic process ultimately depends on the economic profile of the process. Economic assessment in early-stage can guide research and development activities to achieve commercial potential by identifying process bottlenecks. Once these bottlenecks are identified, prioritizing research and development (R&D) efforts to overcome these barriers has the greatest impact on the process. Therefore, such an evaluation can guarantee efficient utilization of limited resources to attain competitiveness in an industrial environment [2]. However, compared with chemical process, the data and modeling for unit operation of biomanufacturing are insufficient. Increasing awareness for economic analysis of biotechnology has a profound and lasting significance for the biomanufacturing development [3]. Application of economic analysis in decision-making in research and design is a key factor to fulfill the economic requirements for commercial operation [4]. This paper demonstrates an approach of economic analysis of biocatalytic processes and illustrates it using a case study. Using the framework of cost analysis and preliminary Catalysts 2020, 10, 1451; doi:10.3390/catal10121451 www.mdpi.com/journal/catalysts Catalysts 2020, 10, 1451 2 of 8 processCatalysts information, 2020, 10, x FOR economicPEER REVIEW assessments of two multi-enzyme system for chiral amine production2 of 9 were studied, namely, transamination process and reductive amination by transaminase and amine dehydrogenasestudied, namely, system, transamination respectively process [5]. Amine and dehydrogenasesreductive amination dependent by transaminase on NADH canand catalyzeamine thedehydrogenase direct reductive system, amination respectively of ketones[5]. Amine with dehydrogenases high enantioselectivity. dependent Asymmetric on NADH can synthesis catalyze of the direct reductive amination of ketones with high enantioselectivity. Asymmetric synthesis of chiral chiral amines by amine dehydrogenases is an ideal route for chiral amines production coupled with a amines by amine dehydrogenases is an ideal route for chiral amines production coupled with a cofactor recycling system with the consumption of an inexpensive agent, such as free ammonia [6]. cofactor recycling system with the consumption of an inexpensive agent, such as free ammonia [6]. Multi-enzyme biosynthesis of chiral amine based on amine dehydrogenases is with the advantages of Multi-enzyme biosynthesis of chiral amine based on amine dehydrogenases is with the advantages simple reaction system and easy co-enzyme regeneration [7,8]. of simple reaction system and easy co-enzyme regeneration [7,8]. 2. Results and Discussion 2. Results and Discussion General assumptions were made as a plant to produce 600 kg (S)-α-methylbenzylamine (MBA) General assumptions were made as a plant to produce 600 kg (S)-α-methylbenzylamine (MBA) (99% ee) per year at concentrations by commercially available transaminases. The plant uses a 2 m3 (99% ee) per year at concentrations by commercially available transaminases. The plant uses a 2 m3 reactor and operates with a 50-h process cycle time. reactor and operates with a 50-h process cycle time. Assume that the equipment cost and waste disposal cost are the same, the reaction time of the Assume that the equipment cost and waste disposal cost are the same, the reaction time of the reductive amination is 48 h, and the reaction time of the transamination is 50 h, which can be roughly reductive amination is 48 h, and the reaction time of the transamination is 50 h, which can be roughly regarded as the same time. Therefore, the difference in total cost lies in the price of raw materials and regarded as the same time. Therefore, the difference in total cost lies in the price of raw materials and the enzyme activity of the reaction. the enzyme activity of the reaction. 2.1. Multi-Enzyme System of Transamination 2.1. Multi-Enzyme System of Transamination Biosynthesis of chiral amines from their corresponding ketones by a multi-enzyme system, Biosynthesis of chiral amines from their corresponding ketones by a multi-enzyme system, which consists of transaminases, glucose dehydrogenase, and lactate dehydrogenase with cofactor which consists of transaminases, glucose dehydrogenase, and lactate dehydrogenase with cofactor regeneration, is selected as a case study (Figure1). The amine donor is alanine. The generated pyruvate regeneration, is selected as a case study (Figure 1). The amine donor is alanine. The generated ispyruvate reduced intois reduced L-lactate into by L-lactate lactate dehydrogenase by lactate dehydrogenase (LDH), which (LDH), eliminates which pyruvate eliminates inhibition pyruvate to theinhibition transaminase to the transaminase and lifts the and reaction lifts the equilibrium. reaction equilibrium. NADH regeneration NADH regeneration is achieved is achieved by glucose by dehydrogenaseglucose dehydrogenase (GDH). Ion-exchange (GDH). Ion-exchange resin is applied resin for is inapplied situ product for in removal.situ product The desiredremoval. product The isdesired separated product with ais moreseparated than with 90% yielda more [5 than]. 90% yield [5]. FigureFigure 1. 1.Multi-enzyme Multi-enzyme catalyzedcatalyzed transamination [5]. [5]. TheThe bioprocess bioprocess shown shown inin FigureFigure2 2is is as as follows: follows: (1)(1) bioconversionbioconversion of the ketone to to amine amine and and cofactorcofactor regeneration, regeneration, (2) (2) separation separation ofof thethe ion-exchangeion-exchange resin resin from from the the reaction reaction mixture mixture by by filtration, filtration, andand (3) (3) isolation isolation and and purification purification of of productproduct amine.amine. Catalysts 2020, 10, 1451 3 of 8 Catalysts 2020, 10, x FOR PEER REVIEW 3 of 9 Figure 2. Overview of the biocatalytic transamination process. Figure 2. Overview of the biocatalytic transamination process. Reaction condition: 100 mM potassium phosphate buffer (pH 7.5), 1 g/L NAD+ (Nicotinamide Reaction condition: 100 mM potassium phosphate buffer (pH 7.5), 1 g/L NAD+ (Nicotinamide adenine dinucleotide), 0.5 g/L pyridoxal-5-phosphate, 90 g/L glucose, 90 g/L alanine, 50 g/L adenine dinucleotide), 0.5 g/L pyridoxal-5-phosphate, 90 g/L glucose, 90 g/L alanine, 50 g/L acetophenone, 1 g/L glucose dehydrogenase (GDH), 1 g/L lactate dehydrogenase (LDH), 5 g/L acetophenone, 1 g/L glucose dehydrogenase (GDH), 1 g/L lactate dehydrogenase (LDH), 5 g/L transaminase (ATA), and 200 g/L ion-exchange resin (Amberlite XAD 1180). Reactions were run at transaminase (ATA), and 200 g/L ion-exchange resin (Amberlite XAD 1180). Reactions were run at 30 ◦C in a Multimax reactor system with overhead mechanical stirring at 400 rpm. 30 °C in a Multimax reactor system with overhead mechanical stirring at 400 rpm. Route cost analysis: Route cost analysis: The cost list of the two synthetic routes is shown in Tables 1–8. See Tables1 and2: The cost list of the two synthetic routes is shown in Tables 1–8: Table 1. Raw material costs per batch of transamination process. Table 1. Raw material costs per batch of transamination process. Concentration Raw Materials Weight (kg/Batch) Unit Price a ($/Kg) Cost ($) Concentration(g/L) Weight Unit Price a Raw Materials Cost ($) Transaminase (ATA-113) 5(g/L) 5(kg/Batch) 50,000($/Kg) 250,000 Lactate
Details
-
File Typepdf
-
Upload Time-
-
Content LanguagesEnglish
-
Upload UserAnonymous/Not logged-in
-
File Pages8 Page
-
File Size-