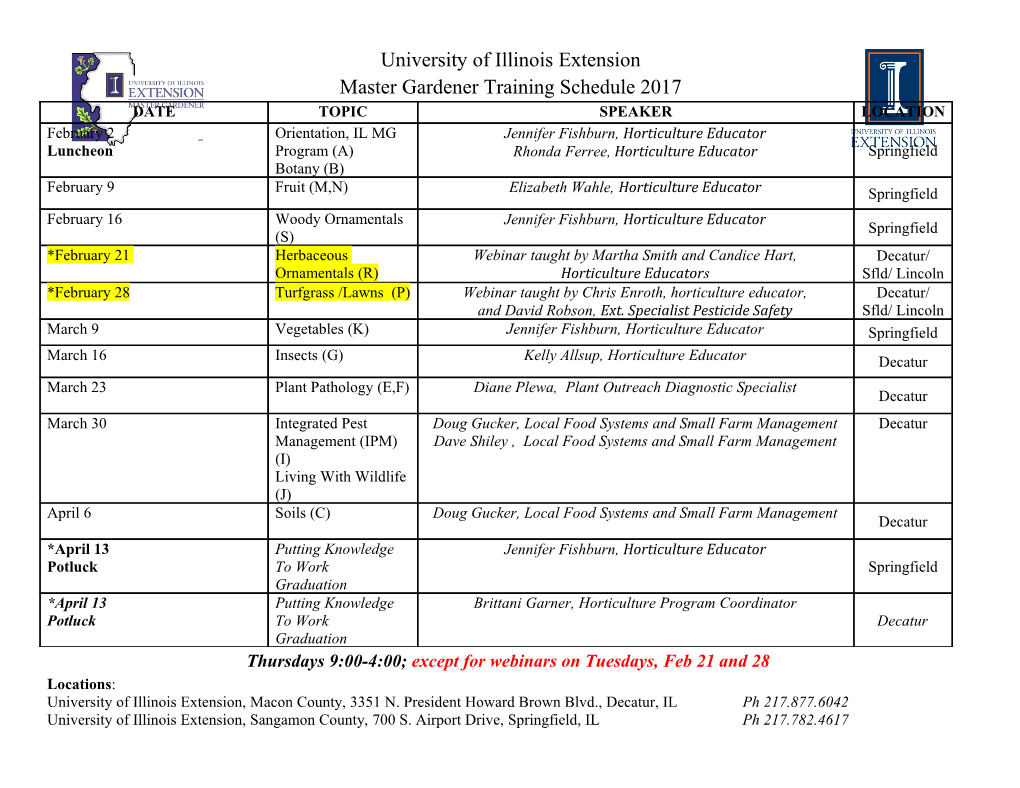
Near-infrared remotely triggered drug-release strategies for cancer treatment Amanda M. Goodmana, Oara Neumannb, Kamilla Nørregaardc, Luke Hendersona, Mi-Ran Choid, Susan E. Clared, and Naomi J. Halasa,b,e,f,1 aDepartment of Chemistry, Rice University, Houston, TX 77005; bDepartment of Electrical and Computer Engineering, Rice University, Houston, TX 77005; cThe Niels Bohr Institute, University of Copenhagen, 2100 Copenhagen, Denmark; dDepartment of Surgery, Feinberg School of Medicine, Northwestern University, Chicago, IL 60611; eDepartment of Physics and Astronomy, Rice University, Houston, TX 77005; and fDepartment of Bioengineering, Rice University, Houston, TX 77005 Contributed by Naomi J. Halas, October 6, 2017 (sent for review July 24, 2017; reviewed by Omid C. Farokhzad and Vincent Rotello) Remotely controlled, localized drug delivery is highly desirable for known highly effective drugs that could otherwise induce toxicity potentially minimizing the systemic toxicity induced by the admin- at high systemic doses. istration of typically hydrophobic chemotherapy drugs by conven- A wide range of host molecules have been developed to pro- tional means. Nanoparticle-based drug delivery systems provide a vide specific binding of therapeutic molecules for nanoparticle- highly promising approach for localized drug delivery, and are an based drug delivery (18–21). DNA and proteins are of particular emerging field of interest in cancer treatment. Here, we demon- interest, since they can be readily conjugated for attachment to strate near-IR light-triggered release of two drug molecules from gold nanoparticle surfaces, and their structures can be tailored – – both DNA-based and protein-based hosts that have been conju- for uptake of drug molecules in a host guest manner (22 24). Host-conjugated nanoparticles can provide efficient internali- gated to near-infrared-absorbing Au nanoshells (SiO2 core, Au shell), each forming a light-responsive drug delivery complex. We show zation into cells and provoke less of an immune response than free drug molecules (25–29). For remotely triggered drug de- that, depending upon the drug molecule, the type of host molecule, ’ and the laser illumination method (continuous wave or pulsed la- livery, equally important is the nanoparticle s light-induced drug- release mechanism. Recently we have shown that continuous-wave ser), in vitro light-triggered release can be achieved with both types (CW)-induced light-triggered release requires the bulk tempera- of nanoparticle-based complexes. Two breast cancer drugs, doce- ture of the illuminated nanoparticle to rise above the thiolated taxel and HER2-targeted lapatinib, were delivered to MDA-MB- dsDNA dehybridization temperature to release ssDNA, while light- 231 and SKBR3 (overexpressing HER2) breast cancer cells and induced release using femtosecond near-IR laser pulses breaks the compared with release in noncancerous RAW 264.7 macrophage Au–S bond that binds the DNA to the nanoparticle with no cells. Continuous wave laser-induced release of docetaxel from a measurable bulk temperature increase (30). nanoshell-based DNA host complex showed increased cell death, Here we investigate near-IR light-triggered release of thera- which also coincided with nonspecific cell death from photothermal peutic molecules using two potential host molecules, thiolated heating. Using a femtosecond pulsed laser, lapatinib release from dsDNA and the protein human serum albumin (HSA), conjugated a nanoshell-based human serum albumin protein host complex onto Au NS to form two optically addressable drug delivery com- resulted in increased cancerous cell death while noncancerous con- plexes, NS@DNA and NS@HSA, respectively. These complexes trol cells were unaffected. Both methods provide spatially and play host to two FDA-approved breast cancer drugs: docetaxel temporally localized drug-release strategies that can facilitate high (DTX) and lapatinib (LAP) molecules. DTX is an antimitotic chemo- local concentrations of chemotherapy drugs deliverable at a specific therapy drug that binds to the inside of microtubules preventing treatment site over a specific time window, with the potential for greatly minimized side effects. Significance chemotherapy | cancer | drug release | near-infrared | nanoparticle Gold-based nanoparticles that absorb near-infrared light have shown the potential to selectively target and treat cancer reast cancer is the leading cause of cancer deaths among through highly efficient light-to-heat conversion. This study Bwomen worldwide, accounting for 1.7 million new diagnoses shows that gold-based nanoparticles can be coated with drug- in 2012 (1). Current treatments for breast cancer include a bearing host biomolecules for remotely triggerable release. combination of surgery, radiation, chemotherapy, molecularly Near-infrared light-triggered release of docetaxel from a nanoshell- targeted, and antihormonal therapeutics (2, 3). One promising based DNA host complex, and lapatinib from nanoshell-based approach in cancer treatment is the use of gold-based nano- DNA and human serum albumin host complexes, is demon- ENGINEERING structures, whose strong optical absorption is due to their plasmon strated. There is a strong dependence upon the type of near- resonance, to provide safe and effective light-based therapeutics. infrared illumination––continuous wave or pulsed––specific to Plasmonic nanostructures are advantageous due to their unique the drug-laden host molecules. Localizing drug delivery both optical properties, low toxicity, in vivo stability, and enhanced spatially and temporally by combining nanoshell-based com- tumor uptake (4–10). One approach uses near-infrared (IR) light plexes and pulsed-laser irradiation is a promising strategy for to heat silica core-gold shell nanoshells (NS) photothermally to highly controlled drug delivery that can apply to a myriad of locally ablate tumors, which has been shown to lead to tumor therapeutic applications. remission in mice at rates above 90% (11–13). In another ap- Author contributions: A.M.G., O.N., S.E.C., and N.J.H. designed research; A.M.G., O.N., proach, near-IR light is used to selectively release oligonucleotides K.N., L.H., and M.-R.C. performed research; A.M.G., O.N., M.-R.C., and S.E.C. analyzed and molecules from the nanoparticle surface for gene therapy and data; and A.M.G., O.N., L.H., M.-R.C., S.E.C., and N.J.H. wrote the paper. drug delivery (14–17). This latter approach also has highly Reviewers: O.C.F., Brigham and Women’s Hospital; and V.R., Harvard Medical School. promising potential for cancer therapy, where high local concen- The authors declare no conflict of interest. trations of drugs could be released remotely, on demand, in a Published under the PNAS license. spatially localized region such as the site of a tumor or metastatic 1To whom correspondence should be addressed. Email: [email protected]. disease, while the overall systemic dosage to a patient would re- This article contains supporting information online at www.pnas.org/lookup/suppl/doi:10. main relatively low. This approach could unleash the potential of 1073/pnas.1713137114/-/DCSupplemental. www.pnas.org/cgi/doi/10.1073/pnas.1713137114 PNAS | November 21, 2017 | vol. 114 | no. 47 | 12419–12424 Downloaded by guest on September 24, 2021 Scheme 1. Nanocomplex formation. (A) Formation of NS@DNA@drug nanocomplex by functionaliza- tion of NS with thiolated dsDNA through Au–thiol bonds, followed by attachment of drug molecules. Release triggered by CW irradiation results in dsDNA dehybridization and release of the drug, while pulsed irradiation results in Au–S bond breakage and release of the dsDNA–drug complex. (B)For- mation of NS@HSA@drug by coating NS with HSA, and attachment of drug molecules. Drug release from the protein is triggered by CW or pulsed irradiation. their disassembly, which disrupts cell division (31). LAP is a ty- NS@DNA@drug nanocomplexes are incubated with cells, then il- rosine kinase inhibitor that works by binding to the intracellular luminated with a near-IR laser. The laser wavelength was selected to ATP binding site of the tyrosine kinase domain of HER2 match the NS plasmon resonance in the near-IR spectral water blocking phosphorylation (32). Growth factor receptors, such window (38). To maximize drug-release efficiency, two illumination as HER2, are proteins on the cell surface that signal it to grow sources were investigated: CW laser irradiation and femtosecond and divide: LAP acts to block the HER2 protein, overexpressed on pulsed-laser irradiation. CW illumination results in dehybridization of some forms of breast cancer, from signaling cell growth (33). Near- the DNA and release of the drug, while pulsed irradiation results in IR light-induced intracellular release was performed in MDA-MB- Au–S bond breakage and release of the dsDNA–drug complex. 231 and SKBR3 (overexpresses HER2) breast cancer cells and HSA is the most abundant protein in blood plasma and can compared with release in noncancerous RAW 264.7 macrophage bind highly insoluble drug molecules in its hydrophobic pockets cells. Macrophages were used as the control cells, as they have (39–41). Due to its large size and multiple binding regions, HSA been shown to be able to phagocytose nanoparticles and deliver has the potential to significantly increase the payload capacity of them to metastatic tumors where photothermal therapy can be the nanocomplexes. NS are coated with HSA, which forms a applied (34, 35). Future studies would involve macrophages de- protein corona around the particles, which are subsequently in- livering these particles for targeted in vivo drug release. Cellular cubated with drug molecules (Scheme 1B). The NS@HSA@drug cytotoxicity was compared when drugs were released from the nanocomplexes are subsequently incubated with cells and irradiated NS@DNA and the NS@HSA complexes. Two methods for near- with either a CW or pulsed near-IR laser in the same manner as the IR light-triggered release, CW laser light at 1.5 W incident power NS@DNA@drug nanocomplexes. and pulsed-laser irradiation, where 160-fs near-IR laser pulses at Functionalization of the NS surfaces was verified with 25 mW average power were used.
Details
-
File Typepdf
-
Upload Time-
-
Content LanguagesEnglish
-
Upload UserAnonymous/Not logged-in
-
File Pages6 Page
-
File Size-