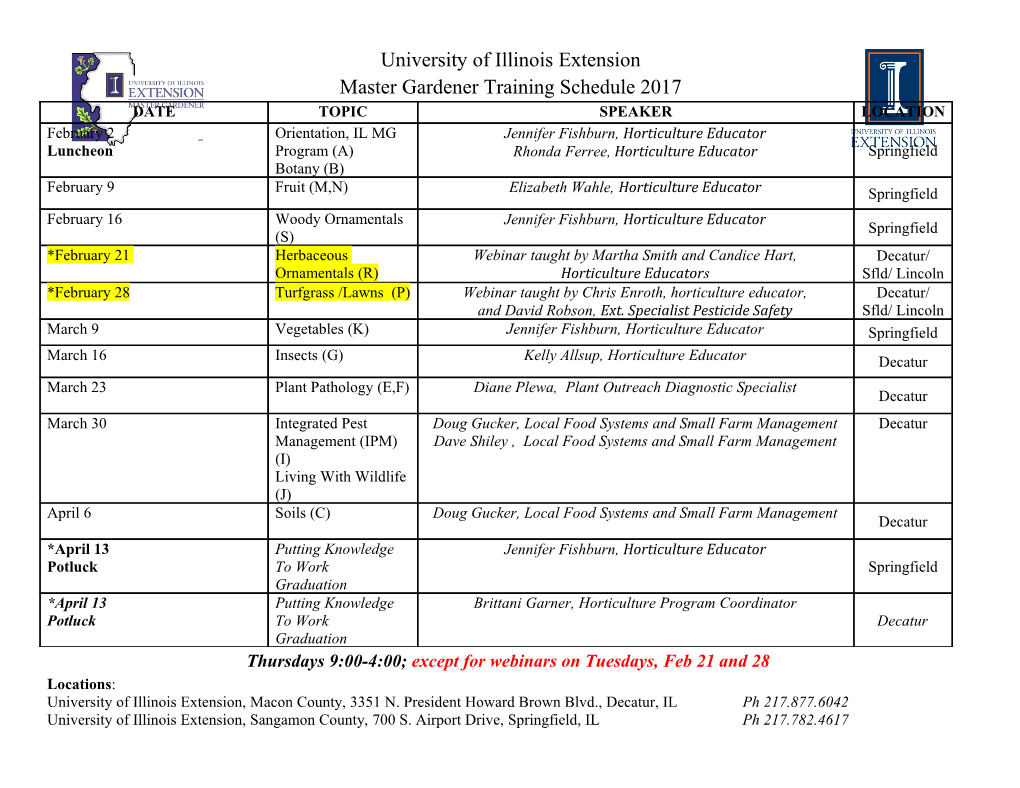
November 15, 2006 Suvranta K. Tripathy Doctor of Philosophy (PhD) Department of Physics of the College of Arts and Sciences Dephasing of excitons and phase coherent photorefractivity in ZnSe quantum wells Professor Hans-Peter Wagner Professor Paul Esposito Professor Leigh Smith Professor Rohana Wijewardhana 1 Dephasing of excitons and phase coherent photorefractivity in ZnSe quantum wells A dissertation submitted to the Division of Research and Advanced Studies of the University of Cincinnati In partial fulfillment of the requirements for the degree of DOCTORATE OF PHILOSOPHY (PhD) In the Department of Physics of the College of Arts and Sciences 2006 By Suvranta K. Tripathy M.S. (Physics), Utkal University, India, 1999 Committee chair: Professor Hans-Peter Wagner 1 Abstract Spectrally resolved and time-integrated degenerate four-wave mixing (FWM) experiments with ultra-short pulses have been used to investigate the relaxation times and coherent interactions of excitons in the Boltzmann and in the quantum kinetic regime in ZnSe single quantum wells. Three-pulse FWM experiments using 100 fs pulses in a 10 nm thick ZnSe/ZnMgSe single quantum well reveal nearly equal zero-density dephasing rates for coherences between σ + and σ − heavy- and/or light-hole exciton states as compared to the dephasing rate of the interband coherence between these states and the ground state. The measurements also demonstrate that exciton–exciton scattering destroys the phase coherence between heavy- and light-hole excitons about three times more effectively than the coherence between σ + and σ + heavy-hole excitons. The different coherent nonlinear signals obtained in a three-beam FWM experiment are explained and reproduced by numerical calculations of the optical Bloch equations for a ten-level model including excitation induced dephasing (EID). Two-beam FWM experiments using 30 fs pulses on a 10 nm ZnSe quantum well reveal marked quantum beats and a pronounced non-exponential decay for delay times smaller ~500 fs. The pronounced peak at pulse overlap is attributed to rapid destructive interference of the created polarization and to spin dependent quantum kinetic effects caused by the transient formation of an electron-hole ( eh ) pair grating. The three-beam FWM trace shows a bi-exponential decay which is attributed to a nonlinear polarization that is caused by the interaction of polarization k3 with both an exciton grating and an eh -pair grating. Model calculations using the OBEs of a two level system that includes 2 EID at both types of density grating provide information about exciton formation processes involved. Model calculations assuming geminate exciton formation reveal an γ −1 = exciton formation time of R 5 ps . Intensity dependent experiments show exciton- β = ± -1 2 eh -pair scattering parameter of X −eh 90 20 s cm . We further observe coherent exciton-LO-phonon polarons in the FWM spectrum of a 3 nm ZnSe single quantum well using 30 fs light pulses. The formation of the new quasi-particles is attributed to a strong phonon coupling that is caused by strong Fröhlich interaction, a huge exciton binding that exceeds the LO-phonon energy and a close resonance between the 2s exciton state and LO-phonon energy. The rapid decay of these coherent quasiparticles is attributed to the disintegration into zero-phonon excitons and free LO-phonons as well as to the inhomogeneous broadening of LO-phonon energies due to disorder and k-space dispersion A novel phase coherent photorefractive (PCP) effect has been observed in ZnSe single QWs using ultra-short pulses that do not overlap in time and its spectral and thermal dependence have been investigated. The observed PCP effect is attributed to the formation of an electron grating formed by the interference of coherent excitons. The experimental results are reproduced by a phenomenological model that is based on optical Bloch equations for a five-level system. The high efficiency of this PCP detectable at an average power of a few µW makes it attractive for applications in all- optical data processing, optical coherent imaging and optical switch applications. 3 4 To my parents, wife and daughter 5 Acknowledgements I take this opportunity to extend my gratitude to the people whose contribution helped to achieve my doctoral dissertation. I would like to thank all faculty, staff, and students at the department of physics, University of Cincinnati for their support throughout my PhD life. My special thanks go to my thesis advisor Prof. Hans-Peter Wagner, who is solely responsible for completing this challenging research work. His new experimental and theoretical ideas and stimulating discussions, his continuous support and encouragement facilitated me to complete my PhD. It has been a pleasure to work with him and I will always be grateful to him for his guidance that brought me up to this position. I would like to thank Prof. Paul Esposito, Prof. Leigh Smith and Prof. Rohana Wijewardhana for serving on my doctoral committee. I am obliged to Prof. Rohana Wijewardhana who was always available to help me to solve problems and to prepare me for the qualifying examination and also for his support in my graduate career in various ways. I am thankful to Prof. Leigh Smith who always gave the confidence and advice to work hard. We are thankful to Prof. D. Hommel for providing us 3 nm ZnSe QW samples. A special acknowledgement is extended to Dr. Hans-Peter Tranitz for his training in ultra-fast lasers, in the FWM experiment and for his explanations, as well as for being a good friend. I want to extend my gratitude to John Markus, the instrumentation specialist at the Department of Physics for his help at many occasions in the lab work. A big thank to Melody Whitlock (former secretary), Donna Deutenberg, Elle Mengon, Bob Schrott, Mark Ankenbauer, Mark Sabatelli, and John Whitaker. Many thanks to my lab- 6 mates Ajith Desilva, Pradeep Bajracharya, Venkat Gangilenka and Amin Kabir for sharing their talents, ideas and humors and for being a part of my PhD life. I would like to thank all of my teachers from Utkal University especially to Prof. Puspa Khare, Prof. Niranjan Barik, Prof. Lambodar Prasad Singh, Prof. Karmadev Maharana, and Prof. Swapna Mahapatra for their endless encouragement and support to have a carrier in physics. My parents have been my strongest source of inspiration. Their blessing, love and emotional support led me to this success in my life. I am indebted to my brothers and sisters whose constant support helped me to come to this point. I express my admiration and deepest gratitude to my wife Meena for her continuous support and understanding, for her love and sacrifice that led me to achieve my dreams. Also I would like to thank my beautiful daughter Selina for being a part of my life who brought lot of happiness during my PhD life. 7 Thesis outline 1. Introduction …………………………………………………………………………16 2. ZnSe based semiconductor quantum wells ………………………………………..20 2.1. Electronic band structure……………………………………………………......20 2.2. Excitons…………………………………………………………………………22 2.3. Linear and non-linear optical properties…………………………………….......29 2.4. Electro-optical properties and photorefractive effect………………………...…31 3. Dephasing of excitons : Theoretical background …………………………………34 3.1. Dephasing of excitons: Boltzmann regime …………………………………...34 3.1.1. Principle of four-wave mixing (FWM)………………………………......35 3.1.2. OBE for a homogeneously broadened system………………………...…38 3.1.3. OBE for an inhomogenously broadened system…………………………39 3.1.4. Various FWM processes…………………………………………………41 3.1.5. Exciton-exciton scattering……………………………………………….45 3.1.6. Exciton-LO phonon interaction………………………………………….46 3.2. Extended OBEs: Ten level model …………………………………………….47 3.3. Brief introduction into quantum kinetics ……………………………………50 4. Description of the experimental setup ……………………………………………..53 4.1. Sub-30 fs laser: Theory and operation ………………………………………..53 4.1.1. Laser pulses………………………………………………………………53 4.1.2. Group velocity dispersion (GVD) and its compensation………………...54 4.1.3. Principle of mode-locking………………………………………………..56 4.1.4. Sub-20 fs Ti: Sapphire laser design……………………………………...57 4.2. Pulse width measurement: Autocorrelation technique ……………………..61 8 4.3. Four-wave mixing experimental setup ………………………………………..67 5. Four-wave mixing experimental results ...................................................................69 5.1. Study of interband and interexciton coherences ……………………………..69 5.1.1. Three-beam FWM experiments………………………………………….69 5.1.2. Theoretical model………………………………………………………..73 5.1.3. Intensity dependent measurements………………………………………78 5.2. Carrier correlated dephasing of excitons in 10 nm ZnSe QW...……………84 5.2.1. Two-beam experiments…………………………………………………..84 5.2.2. Three-beam experiments………………………………………………....92 5.3. Observation of exciton-LO phonon polarons in a 3 nm ZnSe QW………....98 5.4. Phase coherent photorefractive (PCP) effect ……………………………….108 5.4.1. Two-beam FWM measurements; Observation of PCP……………...…108 5.4.2. Three-beam FWM measurements of PCP effect……………………….115 5.4.3. Spectral dependence of the PCP effect…………………………………120 5.4.4. Temperature dependence of the PCP effect…………………………….126 5.4.5. PCP with cw pump laser………………………………………………..128 6. Summary …………………………………………………………………………...131 7. References ………………………………………………………………………….135 9 List of figures Figure 1: Schematic band structure of ZnSe at the center of the Brillouin Zone showing the angular momentum of valence and conduction band and the heavy-hole band and light-hole band away from the center. 21 Figure 2: Schematic diagram of energy band structure of type-I quantum wells. 25 Figure 3: Schematic diagram of a finite potential well of depth V0 given by equation (10) after [1]. 26 Figure 4: Schematic absorption spectra of a two dimensional semiconductor which shows each step of the step function acquires its own excitonic series, after [1]. 27 Figure 5: The diagram depicts the allowed optical transitions for the HH and LH excitons, after [9]. 28 Figure 6: Parallel and perpendicular geometries of a photorefractive quantum well structure, after [15]. 32 Figure 7: Schematic diagram of a three-beam FWM experiment.
Details
-
File Typepdf
-
Upload Time-
-
Content LanguagesEnglish
-
Upload UserAnonymous/Not logged-in
-
File Pages142 Page
-
File Size-