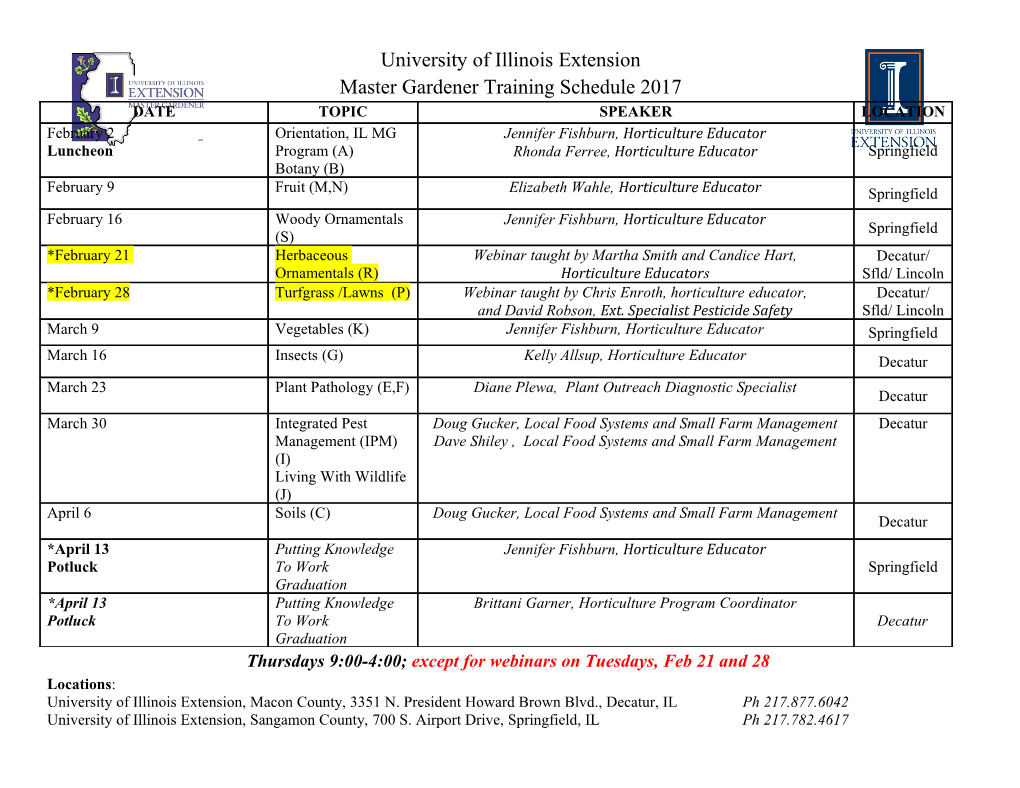
The thermochronological evolution of the northern Gawler Craton and northern Adelaide Rift Complex James William Hall Department of Earth Sciences School of Physical Sciences The University of Adelaide October 2018 Table of contents Abstract v List of Figures vii List of Tables x Declaration xi Journal Articles xii Acknowledgements xiii Introduction and outlines 1 Introduction 1 Thesis outline 4 Chapter 1 – Apatite U-Pb map for the northern Gawler Craton 10 Statement of authorship 11 Abstract 13 1.1 Introduction 13 1.2 Geological setting 14 1.3 Methodology 19 1.4 Results 20 1.4.1 Nawa Domain 20 1.4.2 Coober Pedy Ridge & Mt. Woods Inlier 21 1.4.3 Christie Domain 26 1.4.4 Fowler Domain 28 1.4.5 Wilgena & Nuyts domains 29 1.5 Discussion 31 1.5.1 Palaeoproterozoic cooling 31 1.5.2 Mesoproterozoic cooling 32 1.5.3 Comparisons to pre-existing 40Ar-39Ar datasets and interpolation maps 34 1.6 Conclusions 35 1.7Acknowledgements 36 1.8 References 36 Chapter 2 – Thermal history of the northern Olympic Domain 41 Statement of authorship 42 Abstract 44 2.1 Introduction 44 2.2 Geological setting 45 2.2.1 Gawler Craton 45 2.2.2 Olympic Domain 45 2.2.3 Pandurra Formation, Stuart Shelf and Delamerian Orogeny 46 2.2.4 Post-Delamerian tectonics 48 i 2.2.5 Current regional geothermal gradient 48 2.3 Methodology 49 2.3.1 Rock sampling strategy 49 2.3.2 Apatite thermochronology methods 49 2.3.2.1 Apatite fission track method 52 2.3.2.2 Apatite U-Pb method 52 2.3.3 Potassium feldspar and muscovite 40Ar/39Ar dating 54 2.3.4 (U-Th-Sm)/He analysis 55 2.3.5 Radial plots and modelling 55 2.4 Results 59 2.4.1 Apatite U-Pb age data 59 2.4.2 40Ar/39Ar data 60 2.4.3 Apatite fission track, zircon (U-Th-Sm)/He and apatite (U-Th-Sm)/He data 62 2.4.4 Low-temperature thermochronological modelling 64 2.5 Discussion 65 2.5.1 Proterozoic 65 2.5.2 Palaeozoic 66 2.5.3 Mesozoic 67 2.5.4 Thermal anomalies within the Olympic Domain 69 2.5.5 Comparisons with neighbouring regions 70 2.6 Conclusions 70 2.7 Acknowledgements 71 2.8 References 71 Chapter 3 – Fault reactivation at the northern margin of the Gawler Craton 78 Statement of authorship 79 Abstract 81 3.1 Introduction 81 3.2 Geological setting 82 3.2.1 Gawler Craton 82 3.2.2 Karari Shear Zone 83 3.2.3 Phanerozoic tectonics 83 3.2.4 Offier and Arckaringa Basins 84 3.3 Methodology 84 3.3.1 Apatite fission track dating 84 3.3.2 Radial plots and thermal history modelling 87 3.4 Results 87 3.4.1 Mt. Woods Inlier 87 3.4.2 Nawa Domain 88 3.4.3 Coober Pedy Ridge and Christie Domain 91 3.4.4 Fowler Domain 95 ii 3.4.5 Thermal history modelling 96 3.5 Discussion 96 3.6 Conclusions 99 3.7 Acknowledgements 99 3.8 References 99 Chapter 4 - Constraining (hydro)thermal activity within the Willouran Ranges 105 Statement of authorship 106 Abstract 108 4.1 Introduction 108 4.2 Geological Setting 109 4.2.1 Stratigraphy of the Adelaide Rift Complex 109 4.2.2 Phanerozoic tectonics and hydrothermal activity 109 4.2.3 Domain sub-division 111 4.3 Methodology 114 4.3.1 Apatite fission track analysis 114 4.3.2 Apatite U-Pb analysis 114 4.3.3 Time-temperature models 115 4.4 Results 115 4.4.1 West Mount Domain 115 4.4.2 Rocky Point Sub-Domain and Berlina Domain 115 4.4.3 Kingston and Trial Hole Domains 116 4.4.4 Time-temperature models 125 4.5 Discussion 125 4.5.1 Regional cooling 125 4.5.2 Hydrothermally reset data 126 4.5.3 Comparisons with surrounding regions 127 4.6 Conclusions 128 4.7 Acknowledgements 129 4.8 References 129 Chapter 5 – Exhumation of the Peake and Denison Inliers 133 Statement of authorship 134 Abstract 136 5.1 Introduction 136 5.2 Geological Setting 138 5.3 Methodology 142 5.3.1 Apatite fission track dating 142 5.3.2 Apatite (U–Th–Sm)/He (AHe) and zircon (U–Th–Sm)/He (ZHe) dating 143 5.3.3 Titanite U-Pb dating 143 5.3.4 Radial plots and thermal history modelling 143 iii 5.4 Results 145 5.4.1 Apatite fission track data 145 5.4.2 (U–Th–Sm)/He thermochronology 147 5.4.3 Titanite U-Pb dating 148 5.4.4 Thermal history modelling 149 5.5 Discussion 151 5.5.1 Late Cambrian–Early Carboniferous 152 5.5.2 Middle Carboniferous–Middle Triassic 154 5.5.3 Mesozoic–Cenozoic 154 5.5.4 Comparison with exhumation studies within the Flinders Ranges 155 5.6 Conclusions 156 5.7 Acknowledgements 156 5.8 References 156 Summary and conclusions 163 References 166 Appendices 169 Appendix 1 169 iv Abstract Cratons preserve information about the deformation history of the surrounding terrains and the thermal history of mineral deposits. This thesis aims to constrain the effects on the Gawler Craton of various deformation events from surrounding terrains and the thermal evolution of mineralization within the Craton. Multi-method thermochronology was applied to samples throughout the northern Gawler Craton and Willouran Ranges of the Adelaide Rift Complex to unravel the thermal evolution of the northern Gawler Craton and Willouran Ranges. The subsequent thermal histories are compared and the differences between them highlighted. Apatite U-Pb data reveal distinct spatial patterns within the high temperature cooling history of the Gawler Craton. These spatial patterns correspond to the thermal response of a region to different magmatic and metamorphic events. The eastern Gawler Craton cooled to mid-crustal temperatures from high temperatures following magmatism of the ~1850 Ma Donington Suite and ~1590 Ma Hiltaba Suite while the central Gawler Craton cooling following the ~2500 Ma Sleaford and ~1700 Ma Kimban orogenies. The north-western Gawler Craton preserved evidence for deformation that post-dates cooling relating to magmatism with ages relating to the amalgamation of western and southern Australia at ~1300 Ma. In addition to the apatite U-Pb data, muscovite 39Ar/40Ar preserve post magmatic cooling within the Olympic Domain of the Hiltaba Suite at ~1530 Ma within the Olympic Domain. A second hydrothermally altered muscovite 39Ar/40Ar age from the same sample is recorded at ~1380 Ma. Potassium feldspar 39Ar/40Ar data from numerous samples all preserve altered spectra during the Neoproterozoic which corresponds to the deposition of the Adelaide Rift Complex. The region first cooled to low temperatures (<200 °C) at ~1000 Ma before minor reheating during the Neoproterozoic. The main low temperature regional cooling period is preserved at ~430 – 350 Ma and is interpreted to be caused by deformation relating to the 450 – 300 Ma Alice Springs Orogeny. Younger apatite fission track ages are only preserved in close proximity to major Iron- Oxide-Copper-Gold (IOCG) deposits and form a young thermal corridor linking the IOCG deposits. Apatite fission track data along the Karari Shear Zone of the northern Gawler Craton preserves evidence for regional Neoproterozoic cooling and a deformation event during the Carboniferous. The Nawa Domain preserves Carboniferous apatite fission track ages, whereas the Christie Domain preserves older Neoproterozoic – Cambrian ages. This is interpreted to be resultant from southward compression from the Alice Springs Orogeny and caused the Nawa Domain, to the north of the Karari Shear Zone, to thrust over the Christie Domain to the south. Additionally, this thrusting is interpreted to have ceased deposition of the Officer Basin sediments, which overlies the rocks to the north of the Karari Shear Zone. The deposition of the ~300 Ma Arckaringa Basin sediments indicates that once the compressional event stopped, deposition recommenced in the region. East of the Torrens Hinge Zone, apatite fission track analysis from sedimentary samples within the Willouran Ranges revealed cooling ranging from 550 to 100 Ma. U-Pb data records either detrital v Proterozoic ages or ages at ~250 Ma. This younger age is interpreted to be caused by hydrothermal activity located proximally to the major fault zones in the region. Samples which preserve this younger U-Pb age also preserve apatite fission track ages of ~100 Ma that is interpreted to be cooling. This hydrothermal activity correlates well with hydrothermal activity in the Mt. Painter Inlier, a region of established high heat flow ~150 km to the east. This hints toward a broader zone of hydrothermal activity related cooling during this time. Regional deformation events are consistently present throughout the study region, yet local thermal histories contain inconsistencies. These inconsistencies can be accounted for by differing key geological factors which are unique to each region. The thickness of any overlying sedimentary packages and the geothermal gradient are greatly important at any location as they considerably influence the local thermal histories. Major structures around the rim of the craton tend to control the thermal histories at both high and low temperatures, however, their orientation within the stress regime is important. The prior thermal history of a region can be reset and overprinted by hydrothermal activity as has been recorded in this study for regions within the Olympic Domain and the northern Adelaide Rift Complex. vi List of figures Chapter 1 Fig. 1.1 Simplified geological map of the Gawler Craton 15 Fig. 1.2 Simplified time-space plot of the Gawler Craton 17 Fig.
Details
-
File Typepdf
-
Upload Time-
-
Content LanguagesEnglish
-
Upload UserAnonymous/Not logged-in
-
File Pages255 Page
-
File Size-