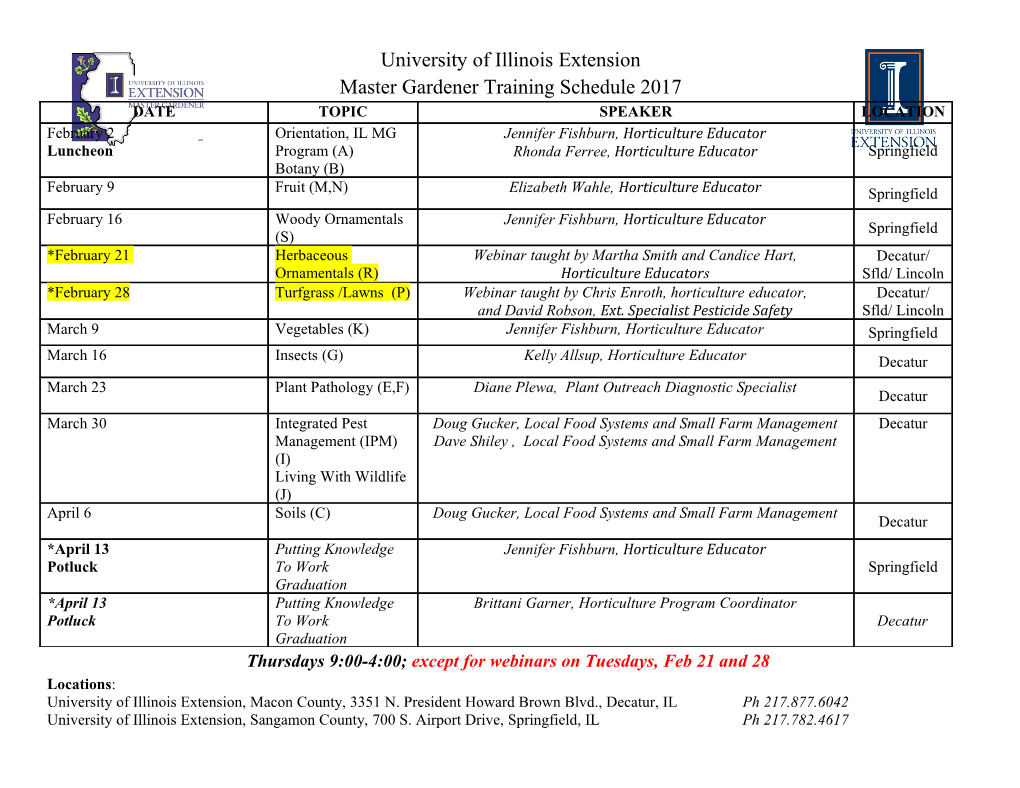
REVIEW ARTICLE NPG Asia Mater. 2(2), 69–78 (2010) doi: 10.1038/asiamat.2010.44 Organic heterostructures in organic fi eld-eff ect transistors Haibo Wang and Donghang Yan* Changchun Institute of Applied Chemistry, China A heterojunction is an interface between two semiconductor materials of diff ering energy gap, and inorganic heterojunctions have been studied as the basis of electronic devices for over half a century. Organic heterojunctions have also been successfully applied in organic electronic devices over the past two decades. However, a theoretical understanding comparable to that of inorganic heterojunctions has yet to be developed for organic heterojunctions. Organic heterojunctions have been drawing increasing attention following the discovery of high conductivity in organic heterojunction transistors constructed with active layers of p-type and n-type thin crystalline fi lms. In contrast with the depletion layers that form in inorganic heterojunctions, electron- and hole-accumulation layers have been observed on both sides of organic heterojunction interfaces. Heterojunction fi lms with high conductivity have been used as charge injection buff er layers and as a connecting unit for tandem diodes. Ambipolar transistors and light-emitting transistors have also been realized using organic heterojunction fi lms as active layers. This review highlights the organic heterojunction eff ect and the application of organic heterostructures in organic fi eld-eff ect transistors. The category of heterojunction, including organic and inorganic semiconductor heterojunctions, is given on the basis of the work function of the constituent materials, and it is shown that a rich variety of organic heterojunctions are possible based on the formation of molecular pairs. onsiderable eff ort has been devoted to the development of or semi-crystalline organic semiconductors [7–9]. Various models have low-cost, fl exible, large-area organic electronics for consumer been proposed to predict the alignment of the vacuum level and the C products over the past two decades [1–3]. Organic fi eld-eff ect interface dipole in certain organic heterojunctions [8,9], and a depen- transistors (OFETs), important organic electronic devices, are of con- dence of the interface dipole on the molecular orientation has been siderable interesting due to their wide range of potential applications, reported [10]. In crystalline organic semiconductors, the phenomenon including use as display drivers and in identifi cation tags and sensors. of band bending [11,12] has been observed at organic/inorganic and Many methods have been developed to improve OFET performance by organic/organic interfaces, and band transport, in which orbital-derived increasing mobility and the on/off ratio, and by reducing the threshold electronic bands are produced due to the overlap of the π-orbitals of voltage. Th ese improvements have been achieved through the synthesis adjacent molecules, has also been argued [13]. of new organic semiconductor materials, improving the device structure, Th e recent discovery of high conductivity in heterojunction transis- controlling the deposition of crystalline organic fi lms and adopting tors constructed using thin crystalline fi lms of p-type copper phtha- various organic heterostructures. Recently, OFETs exhibiting mobility locyanine (CuPc) and n-type copper-hexadecafl uoro-phthalocyanine of the same order of magnitude as that of amorphous silicon FETs have (F16CuPc) as active layers has stimulated interest in organic hetero- been successfully demonstrated. junctions [14–16]. Electron- and hole-accumulation layers have been Organic heterostructures have been used in organic light-emitting observed in these devices on both sides of the organic heterojunction diodes (OLEDs) and organic photovoltaic (OPV) cells to improve interface, which could give rise to interactions that lead to carrier device performance. In a typical double-layer OLED structure [4], redistribution and band bending. In this article, we review the organic the organic heterojunction reduces the onset voltage and improves the heterojunction eff ect and the application of organic heterojunctions illumination effi ciency. Organic heterojunctions have also been used to in OFETs. We show the dependence of the ambipolar transport on improve the power conversion effi ciency of OPV cells by an order of fi lm morphology and device performance in organic heterojunction magnitude over single-layer cells [5]. Ambipolar OFETs, which require transistors, and discuss the application of bulk heterostructures in that both electrons and holes be accumulated and transported in the OFETs. Th e ambipolar transport behavior of organic heterojunctions device channel depending on the applied voltage, were fi rst realized by presents the possibility of fabricating OLED FETs with high quantum introducing organic heterostructures as active layers [6]. It is therefore effi ciency, and a transistor structure for such applications is proposed. clear that organic heterostructures have an important role in the con- Th e application of organic heterostructures as a buff er layer to improve tinued development of organic electronic devices. Th e introduction of the contact between organic layers and metal electrodes is also discussed. organic heterostructures has signifi cantly improved device performance Charge transport in organic semiconductors is infl uenced by many and allowed new functions in many applications, and so understanding factors — the present review emphasizes the use of intentionally doped the eff ects of the organic heterostructure is desirable and necessary. n- and p-type organic semiconductors, and primarily considers organic Th ere has been considerable focus on understanding the interfacial heterojunctions composed of crystalline organic fi lms displaying band electronic structures of organic heterostructure consisting of amorphous transport behavior. *Corresponding author. Email: [email protected] State Key Laboratory of Polymer Chemistry and Physics, Changchun Institute of Applied Chemistry, Chinese Academy of Sciences, Changchun 130022, China Vol. 2 April 2010 | NPG ASIA MATERIALS | www.natureasia.com/asia-materials 69 © 2010 Tokyo Institute of Technology H. B. Wang and D. H. Yan a V =0 V c 1.5 GS 0.2 0 Changing thickness A) +10 ) μ –40 0.1 10 nm 1/2 ( –1 1.0 0 A) F16CuPc(3nm)/CuPc(10nm) F16CuPc(10nm) A DS –50 μ I 0.0 –3 –10 F16CuPc(10nm)/CuPc(5nm) 5 nm –2 A F16CuPc(10nm)/CuPc(10nm) –0.1 (10 F CuPc 0.5 F CuPc(10nm)/CuPc(20nm) CuPc –3 –30 16 Au 1/2 16 DS I Current ( Current F16CuPc Au Au –0.2 F16CuPc –4 –50 CuPc CuPc ITO SiO /Si Single CuPc Insulator –0.3 2 Drain current Drain current 0.0 Glass –5 CuPc/F16CuPc Gate –4 –2 0 2 4 6 8 10 –0.4 V –50–40 –30 –20 –10 0 –2 –1 0 1 2 Gate voltage GS (V) Drain voltage VDS (V) Voltage (V) b Figure 2. Transfer characteristics of F16CuPc/CuPc heterojunction transistors of –8 iii ii Au Au 10 i,iv various thicknesses. These devices show similar electron mobility, and an estimated Au Au F CuPc 16 10–9 hole-accumulation thickness of about 10 nm. Right panel is a schematic of the CuPc CuPc device showing the charge distribution in the double-layer heterojunction film. Glass Glass 10–10 (i) (ii) Modifi ed after Ref. 15, reproduced with permission (© 2006 Elsevier). 10–11 E F Current (A) Current –12 AuF16CuPc Au Au Au 10 F16CuPc CuPc CuPc:F CuPc CuPc 16 –13 Glass Glass 10 source–drain current of the CuPc/F16CuPc transistor is three orders of –60 –40 –20 0 20 40 60 (iii) (iv) Voltage (V) magnitude higher than that of the single-CuPc device under zero gate voltage, indicating that a conducting channel exists in the heterojunc- tion transistor. Furthermore, the threshold voltage is –17 V in the single- Figure 1. Device structures and current–voltage characteristics of CuPc/F16CuPc heterojunction transistors and diodes. (a) Output characteristics of a CuPc/F16CuPc CuPc device and +19 V in the heterojunction device, which also implies heterojunction transistor and a single-component CuPc transistor. The hetero- a change in the mode of device operation. junction transistor operates in hole normally-on mode, which originates from the Th e charge-carrier type in the conducting channel for the normally- heterojunction eff ect of CuPc and F CuPc. Inset shows a schematic of the hetero- 16 on CuPc/F16CuPc heterojunction transistor is dependent on the junction transistor, with mobile free electrons and holes shown in the n- and p-type bottom-layer semiconductor (organic layer near the insulator). When semiconductors, respectively. Modifi ed after Ref. 14, reproduced with permission the bottom-layer semiconductor is p-type CuPc, CuPc/F16CuPc (© 2005 AIP). (b) Device configuration for planar heterojunction diodes and cor- heterojunction transistors operate in hole normally-on mode, whereas responding current–voltage curves: (i) single-CuPc device, (ii) CuPc/F16CuPc diode electron normally-on operation mode is obtained when the bottom- with top-contact configuration, (iii) CuPc/F16CuPc diode with sandwich-contact layer semiconductor is an n-type F16CuPc semiconductor. Th ese results confi guration and (iv) device with a layer of blended CuPc and 16F CuPc. Inset shows a schematic of the predicted interfacial electronic structure. Modifi ed after Ref. 14, demonstrate the abundance of mobile free electrons and holes in the reproduced with permission (© 2005 AIP). (c) Current–voltage characteristic for a F16CuPc and CuPc layers, which is the origin of the normally-on CuPc/F16CuPc heterojunction diode. The device shows a reverse-rectifying prop- characteristic (Figure 1(a), inset). Charge accumulation can lead to erty, which is opposite to that of conventional inorganic p–n junction diodes. Inset upward band bending in p-type CuPc and downward band bending shows the corresponding device confi guration. Modifi ed after Ref. 15, reproduced in n-type F16CuPc from the bulk to the interface (Figure 1(b), inset), with permission (© 2006 Elsevier). which is diff erent to the case for a conventional inorganic p–n junction.
Details
-
File Typepdf
-
Upload Time-
-
Content LanguagesEnglish
-
Upload UserAnonymous/Not logged-in
-
File Pages10 Page
-
File Size-